`.
`Ž
`
`Research report
`Event-related desynchronization in the alpha band and the processing of
`semantic information
`W. Klimesch ), M. Doppelmayr, T. Pachinger, H. Russegger
`Department of Physiological Psychology, UniÕersity of Salzburg, Hellbrunnerstr. 34, A-5020 Salzburg, Austria
`Accepted 30 April 1997
`
`Abstract
`
`The hypothesis was tested whether event-related power shifts in the upper alpha band are specifically related to semantic memory
`processes. In Expt. 1 subjects had to judge whether pairs of sequentially presented words W1-W2 were semantically congruent. In the
`Ž
`.
`following experiments subjects were presented the W1 words of Expt. 1 and were asked to perform a free association task in Expt. 2 and
`a cued recall task in Expt. 3. It is assumed that semantic memory demands dominate in Expt. 1, whereas working memory demands
`dominate in Expt. 3 and that Expt. 2 takes an intermediate position with respect to both types of task demands. A significant task-related
`power change that responds selectively to semantic processing demands was found for the upper alpha band and over the left side of the
`scalp. The lower alpha band, on the other hand, most likely reflects unspecific processing demands such as attention. A more general
`interpretation of these findings is that different cognitive processes such as semantic memory, perceptual encoding and attentional
`processes are reflected by band power changes in different and rather narrow frequency bands over localized regions in the brain. q 1997
`Elsevier Science B.V.
`
`Keywords: Lower alpha; Upper alpha; Desynchronization; Semantic memory; Theta; Episodic memory
`
`1. Introduction
`
`The finding that alpha desynchronizes becomes sup-
`Ž
`pressed in response to a variety of different
`tasks is
`.
`known since the early days of EEG research e.g. 2 . It
`Ž
`w x.
`was and still is generally believed that visual or other
`Ž
`.
`Ž
`sensory
`task demands,
`including visual attention
`cf.
`.
`Ž
`24,25 , are the primary factors that lead to a suppression
`x.
`w
`of the alpha rhythm. More recent research, however, has
`revealed a much more complex picture.
`A better understanding of the functional meaning of the
`alpha rhythm was provided by a new method, which
`Pfurtscheller and his coworkers termed ERD event-related
`Ž
`desynchronization 28 . This method allows to calculate
`x.
`w
`the percentage of event-related power changes for different
`frequency bands. One important finding obtained with this
`method is that the degree and topography of desynchro-
`nization show large and reliable differences between the
`lower and upper alpha band. As an example, desynchro-
`
`) Corresponding author. Fax: q43 662 8044 5126; E-mail:
`Wolfgang.Klimesch@sbg.ac.at
`
`0926-6410r97r$17.00 q 1997 Elsevier Science B.V. All rights reserved.
`
`nization in the lower alpha band is topographically
`widespread without any clear localization, whereas in the
`upper alpha band, desynchronization tends to be more
`localized at those areas which play an important role in
`processing of a particular type of task. For a variety of
`motor tasks Pfurtscheller and colleagues e.g. 23,29,30
`Ž
`x.
`w
`have demonstrated that desynchronization in the upper
`alpha band of about 10–12 Hz
`is localized over the
`Ž
`.
`respective area of the motor cortex over the left or right
`side of the scalp. The fact that the two alpha bands show
`strikingly different results supports the proposal that there
`is no single alpha rhythm but instead a variety of different
`alpha frequencies 20,21 .
`w
`x
`The method of ERD and the distinction between differ-
`ent alpha bands also proved useful for the analysis of
`cognitive processes. As an example, a warning signal
`preceding the presentation of an imperative stimulus causes
`a strong short-lasting synchronization followed by a desyn-
`chronization which most interestingly appears in the lower
`alpha band only 13–15 . In Expt. 1 of Klimesch et al.
`w
`x
`x13 , subjects were required to read a series of words. The
`w
`only manipulation was the variation of the time interval
`between the warning signal and the presentation of a word.
`
`Wave Neuroscience EX2004
`PeakLogic v. Wave Neuroscience
`IPR2022-01550
`
`
`
`84
`
`)
`(
`W. Klimesch et al.rCognitiÕe Brain Research 6 1997 83–94
`
`For the upper alpha band only the presentation of a word –
`but not the warning signal – leads to a localized desyn-
`chronization at posterior sites 11,13–15,17,18 . In con-
`w
`x
`trast, the lower alpha band responds to the presentation of
`both,
`the warning signal and the imperative stimulus.
`These and similar results cf. Expt. 2 in Klimesch et al.
`Ž
`have led to suggest the hypothesis that the
`13,15,17
`x.
`w
`lower alpha band is related to general task demands such
`as attention see also Crawford et al. 5 , whereas the
`Ž
`w x.
`upper alpha band is primarily associated with specific task
`demands such as the visual andror semantic processing of
`the imperative stimulus.
`This latter aspect of the suggested hypothesis was tested
`in a more recent experiment which was designed to com-
`pare the effects of episodic memory and semantic process-
`ing demands 16 . In this experiment we used a design that
`w
`x
`already proved useful to distinguish semantic from episodic
`memory processes
`see Expt. 4 in Kroll and Klimesch
`Ž
`x.19 . The experimental design consisted of two parts.
`w
`Subjects first performed a semantic congruency task in
`which they had to judge whether or not the sequentially
`presented words of concept-feature pairs such as ‘‘eagle-
`Ž
`claws’’ or ‘‘pea-huge’’ are semantically congruent. Then,
`.
`without prior warning,
`they were asked to perform an
`episodic recognition task. This was done in an attempt to
`prevent subjects from using semantic encoding strategies
`and thus to increase episodic memory demands. In the
`episodic task, the same concept-feature pairs were pre-
`sented together with new distractors generated by repair-
`Ž
`ing known concept-feature pairs . Now subjects had to
`.
`judge whether or not a particular concept-feature pair was
`already presented during the semantic task. From the re-
`sults found in Kroll and Klimesch 19 we know that the
`w
`x
`episodic task showing longer RTs and a larger percentage
`Ž
`of incorrect responses
`is much more difficult than the
`.
`semantic task. This latter feature of the design is important
`for our central prediction which is that upper alpha desyn-
`chronizes selectively in response to semantic task de-
`mands. When considering the fact that alpha desynchro-
`nizes with increasing task difficulty, we would expect the
`opposite effect, which is a stronger desynchronization
`during the episodic task. Thus, if task difficulty would be
`the only factor which is reflected by an event-related
`decrease in the upper alpha band reflected by an increase
`Ž
`in ERD we would expect the most pronounced increase in
`.
`ERD during the presentation of the feature in the episodic
`task. This, however, is not the case. The results indicate
`that in spite of the fact that the semantic task is easier than
`the episodic task, the upper alpha band shows a signifi-
`cantly stronger desynchronization during the processing of
`the semantic task.
`The present experiment is designed to further test the
`hypothesis whether the upper alpha band is specifically
`related to the processing of semantic information. The
`experimental design consists of three different tasks termed
`Ž
`Expt. 1, 2 and 3 in the following in which semantic
`.
`
`processing demands are varied. The same sample of sub-
`jects was used in all of the three experiments. In a similar
`way as in the semantic task of Klimesch et al. 16 , in
`w
`x
`Expt. 1 subjects had to judge whether pairs of sequentially
`presented words W1-W2 were semantically congruent. In
`Ž
`.
`the following experiments subjects were presented the W1
`words of Expt. 1 and were asked to perform a free
`association task in Expt. 2 and a cued recall task in Expt.
`3.
`
`Semantic memory is pure long-term memory 7 . The
`w x
`knowledge about semantic relationships like ‘‘an eagle has
`claws’’ is known since childhood and represents ‘‘pre-ex-
`perimental knowledge’’. On the other hand, ‘‘experimental
`knowledge’’ is tested in working memory tasks where
`subjects have to retrieve information that was presented
`earlier in the experiment 1 . Thus, semantic memory
`w x
`demands vary from high in the semantic congruency task
`of Expt. 1 to low in the cued recall
`task of Expt. 3.
`Conversely, working memory demands are high in Expt. 3
`and low in Expt. 1. Expt. 2 takes an intermediate position.
`In a similar way as in Expt. 1 semantic relationships may
`be used to give a response. However, in contrast to Expt.
`1, subjects may use their working memory either to gener-
`ate new words or to retrieve the respective W2 words. The
`term ‘‘free association task’’ is used for Expt. 2 because
`subjects were instructed explicitly to report any word that
`comes into their mind.
`Given this description of task demands, the following
`predictions can be tested:
`Ž .i The semantic congruency task of Expt. 1 can be
`carried out only after the presentation of the second word
`of a pair. Thus, for the upper alpha band we expect the
`strongest task-related desynchronization in the poststimu-
`lus period following the presentation of the W2 word.
`Ž .ii
`In contrast to Expt. 1, semantic memory demands
`are low in Expt. 2 and 3. Thus, as compared to the W2
`words of Expt. 1, desynchronization in the upper alpha
`band will be smaller in Expt. 2 and 3.
`.iii
`In all of the three experiments, general processing
`Ž
`demands, such as attention and effort, will increase from
`the beginning to the end of a task. For the lower alpha
`band we,
`thus, expect a gradual, stepwise increase in
`desynchronization from the beginning of a trial until a
`response is given.
`
`2. Materials and methods
`
`2.1. Subjects
`
`Subjects were 12 right-handed students 7 males, 5
`Ž
`females who participated voluntarily in the experiment.
`.
`Their mean age was 23.7 years with a range of 20–31
`years. Handedness was controlled by asking the subjects
`about the hand they use in different tasks such as handwrit-
`ing, throwing a ball, etc. A prospective subject was consid-
`
`
`
`)
`(
`W. Klimesch et al.rCognitiÕe Brain Research 6 1997 83–94
`
`85
`
`ered right-handed if hershe indicated to use the right hand
`for all of these different tasks.
`
`2.2. Materials
`
`A set of 192 feature and concept words was used as
`stimuli. Half of the words belonged to the category ‘‘liv-
`ing’’ birds, fruits, vegetables , the other half to ‘‘non-liv-
`Ž
`.
`ing’’ vehicles, clothes, weapons . For a detailed descrip-
`Ž
`.
`tion of a similar set of stimuli, including word norms, see
`Kroll and Klimesch 19 .
`w
`x
`The words appeared at the center of a computer monitor
`and were 0.7 cm in height. The length of a word with 10
`letters was 5.7 cm. Subjects sat at a distance of 90 cm
`from the monitor.
`
`2.3. Design
`
`In Expt. 1 subjects had to perform a semantic congru-
`ency task on sequentially presented pairs of words W1,
`W2 by responding ‘‘yes’’ to a congruent and ‘‘no’’ to an
`incongruent pair e.g., W1 sclaws, W2 seagle; W1 s
`Ž
`blue, W2 scanary . Subjects were instructed to report as
`.
`quickly as possible but not before a response signal cf.
`Ž
`Fig. 1 was presented. The W1 words are also called
`.
`‘‘feature words’’, whereas the W2 words represent ‘‘con-
`cept words’’. Feature words were paired with concept
`words W2 words
`in a way that half of the pairs were
`Ž
`.
`congruent, the other half incongruent. Subjects were in-
`formed that word pairs representing a ‘‘feature’’ and a
`‘‘concept’’ e.g., ‘‘claws-eagle’’ will be presented. They
`Ž
`.
`were asked to give a positive response if the pair is
`semantically congruent and a negative response if the pair
`is incongruent e.g., ‘‘blue-canary’’ .
`Ž
`.
`In Expt. 2 and 3 only half of the W1 words were
`presented. These were those feature words which in Expt.
`1 were paired with congruent concept words. The experi-
`mental design is shown in Fig. 1.
`In Expt. 2 subjects were asked to carry out a free
`association task. They were instructed to report any word
`that would come into their minds as quickly as possible
`but after a response signal was presented. Subjects knew
`that feature words of Expt. 1 – arranged in a different
`sequence – will be presented. They were asked to avoid
`repetitions, i.e., responding with the same association more
`than once.
`Finally, in Expt. 3 and without prior warning a cued
`recall task was performed. Subjects were informed that
`half of the feature words of Expt. 1 – arranged in a
`different sequence – will be presented again. But now they
`were asked to remember and report that concept word that
`was paired with the respective feature word in the seman-
`tic task of Expt. 1. Again, subjects were asked to wait with
`their response until a response signal appeared on the
`screen.
`
`Fig. 1. In Expt. 1 subjects had to judge whether pairs of sequentially
`presented feature words W1 and concept words W2 were semantically
`Ž
`.
`Ž
`.
`congruent. In Expt. 2 subjects were asked to respond with a free
`association to W1, and in Expt. 3, W1 served as a cue to recall the
`respective concept word W2 . A warning signal WS preceded the
`Ž
`.
`Ž
`.
`presentation of W1. Test intervals t1–t5 represent time periods of 500 ms
`each.
`
`The main reason for using only those feature words
`which were congruent in Expt. 1 was to avoid that the type
`of response in Expt. 1 which represents episodic informa-
`Ž
`tion interferes or interacts with the type of response in
`.
`Expt. 2 and 3 in an uncontrolled way. As an example,
`when trying to remember the corresponding concept word
`in Expt. 3 subjects could try to remember whether a
`presented feature word elicited a yes or no response in
`Expt. 1. A strategy like this would prevent subjects from
`retrieving the semantic relationship between the feature
`and concept word. The use of congruent feature words in
`Expt. 2 and 3 makes it, thus, more likely that a subject’s
`response is based on task relevant semantic instead of
`irrelevant episodic information.
`As indicated by Fig. 1, the EEG is analyzed in time
`intervals of 500 ms each, preceding and following the
`presentation of a word. These time intervals are termed
`t1–t5. Interval t1 represents the prestimulus period. During
`t2 and t4 in Expt. 1 the presented W1 or W2 word is
`Ž
`.
`encoded, whereas in t3 and t5 in Expt. 1 the primary
`Ž
`.
`focus is on the cognitive processing of the respective word.
`Semantic congruency judgments are easy and,
`thus,
`quite fast to perform. As the analysis of reaction times
`.RTs has shown, the average semantic verification time –
`Ž
`as measured from the onset of the W2 word – is less than
`1 s cf. Expt. 2a in Kroll and Klimesch 19 . In addition,
`Ž
`x.
`w
`the results of Expt. 2b in Kroll and Klimesch 19 have
`w
`x
`shown that under otherwise comparable conditions lexi-
`.
`Ž
`cal verification time the time needed to distinguish a word
`Ž
`from a non-word is 677 ms. Earlier performed experi-
`.
`ments 10 have indicated that about 200 ms are needed to
`w
`x
`carry out the type of motor response that was required in
`these tasks i.e., moving the right finger and pressing the
`Ž
`respective response key . This means that
`in the first
`.
`poststimulus interval of 0–500 ms t2 or t4 respectively a
`Ž
`.
`word is lexicographically processed, whereas semantic ver-
`ification or processing takes place primarily during the
`second poststimulus interval
`t3 or t5 respectively .
`Ž
`.
`
`
`
`86
`
`2.4. Procedure
`
`)
`(
`W. Klimesch et al.rCognitiÕe Brain Research 6 1997 83–94
`
`All of the words were presented for 250 ms on a
`computer monitor. In all of the experiments, the length of
`a single trial epoch was 8 s. A brief warning signal 3000
`Ž
`.
`Ž
`Hz, lasting for 250 ms appeared 1000 ms before a W1
`.
`word was presented. The structure of single trials is shown
`in Fig. 1.
`In an attempt to avoid artifacts, subjects were asked to
`maintain fixation by looking at the middle of the screen as
`soon as an acoustic warning signal appeared. As Fig. 1
`illustrates, the response signal question mark appeared
`Ž
`.
`1500 ms after presentation onset of a W2 word in Expt. 1
`Ž
`.
`or a W1 word in Expt. 2 and 3 . This procedure was also
`Ž
`.
`used to avoid response artifacts and to obtain poststimulus
`processing times which are of comparable duration in all
`of the three conditions. This was done even at the risk of a
`slight increase in working memory demands at or after t5
`or t3 respectively.
`Subjects responded verbally by saying ‘‘yes’’ or ‘‘no’’
`in Expt. 1, by reporting an association in Expt. 2 and by
`reporting a concept word in Expt. 3. All of the responses
`were recorded by the experimenter. Each experiment was
`preceded by a series of four training trials.
`
`2.4.1. Apparatus
`EEG signals were amplified by a 32-channel biosignal
`amplifier system frequency response: 0.16–30 Hz , sub-
`Ž
`.
`jected to an anti-aliasing filter bank cut-off frequency: 30
`Ž
`Hz, 110 dBroctave and were then converted to a digital
`.
`format via a 32-channel ArD converter. Sampling rate
`was 128 Hz. The data were processed by the B.E.S.T.
`system of Grossegger and Drbal which is based on a 486
`PC. During data acquisition, EEG signals were displayed
`on-line on a high resolution monitor and stored on disk.
`
`2.4.2. Recordings
`A set of 22 silver electrodes, attached with a glue paste
`to the scalp, was used to record EEG signals. Ten elec-
`trodes were placed according to the international electrode
`10–20 placement system, at F3, F4, C3, C4, T3, T4, P3,
`Ž
`.
`P4, O1 and O2. From the remaining 12 electrodes, four
`electrodes were placed over parieto-occipital areas PO3,
`Ž
`PO1, PO2, PO4 , four electrodes were placed over centro-
`.
`parietal areas CP5, CP1, CP2, CP6 , and four electrodes
`Ž
`.
`were placed over fronto-central areas FC5, FC1, FC2,
`Ž
`FC6 . For the placement of these additional electrodes see,
`.
`e.g., Herrmann et al. 6 .
`w x
`In addition to the 22 electrodes described above, two
`mastoid electrodes termed A1 and A2 were attached to
`Ž
`.
`the left and right ear. Furthermore, the electrooculogram
`EOG was recorded from two pairs of leads in order to
`Ž
`.
`register horizontal and vertical eye movements.
`All data were recorded monopolarly against a common
`reference placed on the nose. In order to eliminate the
`effects of the nose reference as well as other types of
`
`artifacts, the EEG recordings were corrected by subtracting
`the arithmetically averaged mastoid recordings A1 q
`Ž
`.A2 r2 from all of the monopolar recordings.
`All of the epochs in each of the three experiments were
`checked individually for artifacts eye blinks, horizontal
`Ž
`and vertical eye movements, muscle artifacts, etc. by
`.
`visual inspection. Only epochs with a correct yes or no
`response were used for data analysis. The percentage of
`epochs that were excluded from data analysis was 5.9%
`Ži.e., no response to a congruent pair, or yes response to an
`incongruent pair in Expt. 1, 12.9% missing association or
`.
`Ž
`associations reported more than once
`in Expt. 2 and
`.
`12.1% missing response or concept word reported more
`Ž
`than once in Expt. 3.
`.
`
`2.4.3. The calculation of eÕent-related changes in band
`power
`The data for each epoch and each of the 22 channels
`were digitally band-pass filtered, squared in order to
`Ž
`obtain simple power estimates and averaged separately for
`.
`each experimental condition and for each subject. Based
`on these data, event-related changes in band power were
`calculated in using a procedure which was originally pro-
`posed by Pfurtscheller and Aranibar 28 who coined the
`w
`x
`term event-related desynchronization or ERD. ERD is
`defined as the percentage of decrease or increase in band
`power during a test interval as compared to a reference
`interval: ERD % s band power, reference interval y
`wŽ
`.
`band power, test interval r band power, reference inter-
`Ž
`.x
`Ž
`val =100. For a detailed description see e.g. 31 . Note
`.4
`w
`x
`that positive ERD values indicate a state of desynchroniza-
`tion or power suppression. Negative ERD values, on the
`other hand, reflect a state of synchronization or increase in
`band power.
`In the present study, an interval of 1000 ms beginning
`Ž
`2500 ms before and ending 1500 ms before the presenta-
`tion of the feature word was used as reference interval cf.
`.
`Ž
`Fig. 1 . The test intervals consist of time intervals of 500
`.
`ms preceding and following the presentation of a word cf.
`Ž
`the time intervals t1–t5 in Fig. 1 .
`.
`After rejecting artifacts, an average of 147, 56 and 63
`epochs remained for Expt. 1, 2 and 3 respectively.
`
`2.4.4. The indiÕidual determination of frequency bands
`The frequency windows for the theta and alpha bands
`were determined individually for each subject i by using
`mean peak frequency f i of the dominant EEG frequency
`Ž .
`in the alpha band for all recording sites as an anchor point.
`Mean peak frequency f i was calculated over the entire
`Ž .
`epoch of 8 s. In using f i as individual anchor point, four
`Ž .
`different frequency bands with a bandwidth of 2 Hz each
`were defined:
`f i -6 to f i -4 ;
`f i -4 to f i -2 ;
`f i -2
`Ž Ž .
`.
`Ž Ž .
`. Ž Ž .
`.
`Ž Ž .
`. Ž Ž .
`.
`to f i q2 . The ERD was calculated within
`to f i ; f i
`Ž Ž .
`Ž .
`Ž .
`.
`these individually determined frequency bands which are
`termed theta,
`lower-1 alpha,
`lower-2 alpha, and upper
`alpha. The averaged mean frequency f i was 10.0 Hz.
`Ž .
`
`
`
`)
`(
`W. Klimesch et al.rCognitiÕe Brain Research 6 1997 83–94
`
`87
`
`Thus, averaged over the entire sample of 12 subjects, the
`following cut-off points were obtained: 4–6 Hz, 6–8 Hz,
`8–10 Hz, 10–12 Hz.
`With frequency bands of 2 Hz, epochs down to a length
`of 0.5 s can still be analyzed. However, the effects of
`spectral leakage i.e.,
`the influence of neighboring fre-
`Ž
`quency bands becomes the more severe, the shorter the
`.
`epoch length is. One way to compensate for this effect is
`to use a large number of epochs over which power esti-
`mates are averaged. By averaging, the distorting effects of
`neighboring frequency bands decrease and the accuracy of
`power estimates increases. This is for the same reason why
`the accuracy of any estimate increases with increasing
`sample size. Because only the averaged data of at least 56
`Ž
`trials are used for statistical analyses, the reported results
`.
`are based on power estimates that can be considered
`sufficiently reliable.
`With respect to the naming of the frequency bands it
`should be emphasized that the frequency band of 6–8 Hz,
`
`which is termed ‘‘lower-1 alpha’’, shows only a marginal
`overlap with the traditional alpha band of 7–13 Hz. How-
`ever, as the results reported below will show, this fre-
`quency band exhibits effects that are quite similar to the
`lower-2 alpha which ranges from 8 to 10 Hz. As the
`lower-2 alpha band and in contrast to the theta band, the
`lower-1 alpha band desynchronizes with increasing task
`demands. Thus, for simplicity, we use the term lower-1
`alpha even for this slow frequency range.
`This method of adjusting frequency bands individually
`before analyzing ERD is important because of the follow-
`ing two findings:
`i alpha frequency varies as a function
`Ž .
`of several factors such as age, memory performance and
`attentional demands cf. 11,14 and the summary in 8,9 ;
`Ž
`x.
`w
`x
`w
`the frequency of the transition region between a task-
`Ž .ii
`related decrease in band power as an indicator for the
`Ž
`alpha band and a task-related increase in band power as
`.
`Ž
`an indicator for the theta band shows also a tendency to
`.
`vary as a function of alpha frequency see e.g. 18 . It is,
`Ž
`x.
`w
`
`Table 1
`
`Time
`ANOVA results: Expt. 1, semantic task
`df numerator
`4
`Ž
`.
`df denom.
`44
`Ž
`.
`Theta
`3.13
`0.55
`24.32
`0.43
`39.92
`0.58
`5.28
`0.43
`
`Lower-1 alpha
`
`Lower-2 alpha
`
`Upper alpha
`
`F
`
`e
`
`F
`
`e
`
`F
`
`e
`
`F
`
`e
`
`Location
`
`4
`44
`2.00
`0.41
`1.47
`0.57
`4.00
`0.57
`11.67
`0.59
`
`a
`
`b
`
`4
`44
`1.28
`0.46
`1.08
`0.57
`4.97 a
`0.55
`7.31
`0.50
`
`b
`
`4
`44
`2.73
`0.44
`1.16
`0.55
`11.05
`0.60
`23.83
`0.61
`
`b
`
`b
`
`Hemi
`
`1
`11
`0.54
`–
`1.44
`–
`1.51
`–
`2.31
`–
`
`1
`11
`2.18
`–
`0.01
`–
`0.07
`–
`1.51
`–
`
`1
`11
`0.00
`–
`0.91
`–
`1.25
`–
`0.34
`–
`
`T =L
`
`16
`176
`3.62
`0.29
`2.08
`0.13
`3.92
`0.17
`4.60
`0.15
`
`8
`88
`4.38
`0.47
`2.50
`0.42
`2.67
`0.39
`3.04
`0.36
`
`8
`88
`8.35
`0.39
`8.52
`0.34
`3.34
`0.35
`6.19
`0.30
`
`b
`
`a
`
`a
`
`b
`
`a
`
`b
`
`b
`
`a
`
`b
`
`T =H
`
`L =H
`
`T =L =H
`
`4
`44
`0.17
`0.68
`0.10
`0.43
`0.66
`0.40
`7.63
`0.57
`
`2
`22
`3.96
`0.96
`0.64
`0.82
`0.22
`0.60
`2.40
`0.95
`
`2
`22
`0.02
`0.74
`0.55
`0.79
`4.75
`0.83
`2.86
`0.82
`
`b
`
`a
`
`a
`
`4
`44
`0.90
`0.49
`0.26
`0.63
`0.45
`0.44
`3.20
`0.65
`
`4
`44
`0.07
`0.62
`0.89
`0.55
`1.98
`0.60
`3.00
`0.54
`
`4
`44
`1.40
`0.75
`1.71
`0.56
`2.59
`0.62
`4.64
`0.59
`
`a
`
`a
`
`a
`
`b
`
`a
`
`16
`176
`0.95
`0.34
`2.08
`0.32
`2.69
`0.23
`5.15
`0.30
`
`8
`88
`0.72
`0.42
`2.58
`0.40
`2.59
`0.35
`3.40
`0.31
`
`8
`88
`1.59
`0.38
`0.96
`0.51
`1.63
`0.47
`2.51
`0.42
`
`b
`
`b
`
`a
`
`b
`
`a
`
`b
`
`ANOVA results: Expt. 2, free association
`df numerator
`2
`Ž
`.
`df denom.
`22
`Ž
`.
`Theta
`1.41
`0.81
`16.85
`0.65
`12.20 b
`0.78
`4.65
`0.77
`
`Lower-1 alpha
`
`Lower-2 alpha
`
`Upper alpha
`
`F
`
`e
`
`F
`
`e
`
`F
`
`e
`
`F
`
`e
`
`ANOVA results: Expt. 3, cued recall
`df numerator
`2
`Ž
`.
`df denom.
`22
`Ž
`.
`Theta
`1.94
`0.84
`2.73
`0.95
`36.34
`0.89
`2.84
`0.88
`
`Lower-1 alpha
`
`Lower-2 alpha
`
`Upper alpha
`
`F
`
`e
`
`F
`
`e
`
`F
`
`e
`
`F
`
`e
`
`T, time; L, location; H, Hemi recording sites over the left and right sides of the scalp .
`.
`Ž
`a 5%; b 1% level of significance.
`
`
`
`88
`
`)
`(
`W. Klimesch et al.rCognitiÕe Brain Research 6 1997 83–94
`
`as a
`important
`thus,
`to use alpha peak frequency f i
`Ž .
`common reference point for adjusting different frequency
`bands not only for the alpha, but theta range as well.
`
`2.4.5. Statistical analyses
`Three different
`types of ANOVAs were calculated.
`First, 3-factorial ANOVAs were calculated to compare the
`W1 words of congruent pairs with the W1 words of
`incongruent pairs of Expt. 1. Second, 3-factorial ANOVAs
`were calculated for comparisons within each of the three
`experiments. Third, 4-factorial ANOVAs were computed
`to analyze effects that are due to differences between the
`experiments.
`In order to check whether the W1 words in congruent
`and incongruent pairs represent homogeneous subsamples,
`ERD data were subjected to 3-factorial ANOVAs. This
`was done separately for each of the four frequency bands
`and for each of the first three time intervals t1, t2, t3 . The
`Ž
`.
`factors and their levels are: Kong: W1 words of congruent
`pairs versus W1 words of incongruent pairs; Location:
`frontal F3, F4, FC5, FC6 , central C3, C4, FC1, FC2,
`Ž
`.
`Ž
`CP1, CP2 , parietal P3, P4, CP5, CP6 , temporal T3, T4
`.
`Ž
`.
`Ž
`.
`and occipital O1, O2, PO3, PO1, PO2, PO4 leads; and
`Ž
`.
`Hemi: recording sites over the left and right
`l, r side of
`Ž
`.
`the scalp.
`For each of the three experiments and each of the four
`frequency bands, ERD data were subjected to 3-factorial
`ANOVAs. The factors are Time, Location, and Hemi. For
`factor Time the levels are intervals of 500 ms preceding
`and following the presentation of a word t1, t2, t3, t4 and
`Ž
`t5 in Expt. 1; t1, t2 and t3 in Expt. 2 and 3 . The levels of
`.
`Location and Hemi were already described above. The
`results of the respective ANOVAs are summarized in
`Table 1.
`For the analysis of differences between the experiments
`4-factorial ANOVAs were calculated for each frequency
`band. The factors Location, Hemi and Time with only
`Ž
`three levels: t1, t2, and t3 were identical with the respec-
`.
`tive factors of the 3-factorial ANOVAs. The only addi-
`tional factor is Task with three levels: semantic, free
`Ž
`association, and cued recall task .
`.
`The Greenhouse–Geisser procedure was used to com-
`pensate for violations of sphericity or circularity. For
`repeated measurement factors with more than two levels,
`the epsilon factor e and the adjusted tail probabilities
`Ž
`.
`will be reported.
`
`3. Results
`
`3.1. BehaÕioral data
`
`In Expt. 1, an average of 5.9% of the responses were
`incorrect yes response to an incongruent or no response to
`Ž
`a congruent pair . In Expt. 2, in 22.5% of the cases a
`.
`subject associated the correct concept word, in 31.8% of
`
`the cases any concept or feature word that was presented
`in Expt. 1. An average of 32.8% were new associations.
`No association or repeated associations were observed in
`12.8% of the cases. In Expt. 3, the percentage of correctly
`remembered concept words was 29.3%
`
`3.2. Experiment 1
`
`None of the 12 3-factorial ANOVAs which were calcu-
`lated to check for differences between the group of W1
`words of congruent and that of incongruent pairs showed
`significant results at or beyond the 5% level
`in which
`Ž
`.
`factor Kong either as main effect or interaction is in-
`Ž
`.
`volved. This finding demonstrates that the W1 words of
`congruent and incongruent pairs can be considered a ho-
`mogeneous sample. Therefore, the data for congruent and
`incongruent pairs were pooled in all of the analyses re-
`ported below. This finding is important because only those
`W1 words were used in Expt. 2 and 3 which belonged to
`congruent pairs in Expt. 1 see Section 2 .
`Ž
`.
`For the theta band, only one significant result was
`found as the summary of results in Table 1 shows. Inspec-
`tion of the respective means of the significant interaction
`Time =Location reveals that only at occipital recording
`sites and only during t2 stimulus onset of W1 and t4
`Ž
`.
`stimulus onset of W2 , a pronounced synchronization
`Ž
`.
`increase in power was found.
`Ž
`.
`For the lower-1 alpha band the only significant finding
`is a main effect for Time. The respective means are plotted
`in Fig. 2 and show a gradual increase in desynchronization
`´
`from t1 to t5. According to the Scheffe test for pairwise
`comparisons the critical difference at the 5% level is 10.2.
`As compared to t1, the increase in ERD is significant at
`and beyond t3.
`The significant main effect for Time in the lower-2
`alpha band shows a similar result cf. Fig. 2 . In addition,
`Ž
`.
`factor Location indicating that the amount of desynchro-
`Ž
`nization is more pronounced at posterior as compared to
`anterior
`recording sites
`and the interactions Time =
`.
`Location and Time =Location =Hemi reached signifi-
`cance. The 3-way interaction indicates a left hemispheric
`advantage stronger desynchronization over the left side of
`Ž
`the scalp which can be observed during t2–t4 but not in
`.
`Ž
`t1 and which is particularly large at anterior
`including
`Ž
`.
`temporal
`recording sites. For the levels of the significant
`.
`´
`main effect of Time, the Scheffe test for pairwise compar-
`isons yields a critical difference of 10.6 at the 5% level. As
`compared to t1, the increase in ERD is significant at and
`beyond t3.
`In the upper alpha band all but one variance sources
`reached significance. The significant main effect for Time
`´
`is depicted in Fig. 2a. The Scheffe test for pairwise
`comparisons yields a critical difference of 18.9 at the 5%
`level. As indicated by the respective arrow in Fig. 2a, the
`increase in poststimulus ERD is significant at t5 only.
`Because the main effects as well as the three significant
`
`
`
`)
`(
`W. Klimesch et al.rCognitiÕe Brain Research 6 1997 83–94
`
`89
`
`3.3. Experiment 2
`
`The theta band shows two significant interactions, Time
`=Location and Time =Hemi. Inspection of the respective
`means reveal a tendency towards an increase of band
`power synchronization which is most pronounced at oc-
`Ž
`.
`cipital recording sites during t2 cf. Fig. 4a . Hemispheric
`Ž
`.
`differences showing a general tendency of an increase in
`band power in the left hemisphere for all recording sites
`can be observed during t2 and t3.
`As in Expt. 1,
`the only significant finding for the
`lower-1 alpha band is a main effect for Time. Again, the
`respective means as depicted in Fig. 2 show a gradual
`increase in desynchronization from t1 to t3. The Scheffe´
`test for pairwise comparisons yields a critical difference of
`12.5 at the 5% level cf. Fig. 2b .
`Ž
`.
`
`Fig. 2. Event-related desynchronization ERD , reflecting task-related
`.
`Ž
`band power changes in the three experiments and the four frequency
`bands. A significant increase in desynchronization in one of the poststim-
`ulus intervals t2–t5 with respect to the prestimulus level of activity t1 is
`marked by an asterisk. Semantic memory demands are highest in Expt. 1
`but during t4 and t5 only. Note that only the upper alpha band responds
`selectively to this type of task demands.
`
`2-way interactions are included in the 3-way interaction,
`the description of this result which is depicted in Fig. 3
`will suffice to explain all of the remaining significant
`findings. Fig. 3 shows a pronounced increase in desyn-
`chronization which is much stronger over the left hemi-
`Ž
`sphere for frontal, central, temporal, and parietal record-
`.
`ing sites that can be observed primarily during t5 i.e.,
`Ž
`during the processing of the semantic task . Most interest-
`.
`ingly, the increase in desynchronization from t1 to t5 is
`significant for all of the five recording locations over the
`left hemisphere but for none of the right hemisphere. In the
`right hemisphere, a significant increase was found only
`from t1 to t4 cf. the respective arrows in Fig. 3 . The
`Ž
`.
`judgment of a significant increase from t1 to one of the
`poststimulus interval
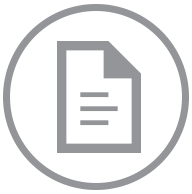
Accessing this document will incur an additional charge of $.
After purchase, you can access this document again without charge.
Accept $ ChargeStill Working On It
This document is taking longer than usual to download. This can happen if we need to contact the court directly to obtain the document and their servers are running slowly.
Give it another minute or two to complete, and then try the refresh button.
A few More Minutes ... Still Working
It can take up to 5 minutes for us to download a document if the court servers are running slowly.
Thank you for your continued patience.
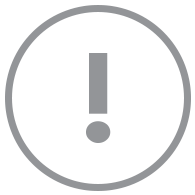
This document could not be displayed.
We could not find this document within its docket. Please go back to the docket page and check the link. If that does not work, go back to the docket and refresh it to pull the newest information.
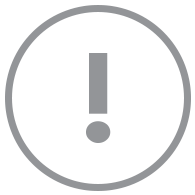
Your account does not support viewing this document.
You need a Paid Account to view this document. Click here to change your account type.
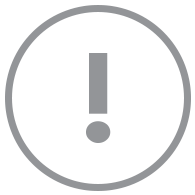
Your account does not support viewing this document.
Set your membership
status to view this document.
With a Docket Alarm membership, you'll
get a whole lot more, including:
- Up-to-date information for this case.
- Email alerts whenever there is an update.
- Full text search for other cases.
- Get email alerts whenever a new case matches your search.
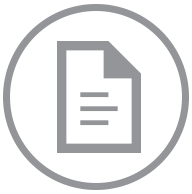
One Moment Please
The filing “” is large (MB) and is being downloaded.
Please refresh this page in a few minutes to see if the filing has been downloaded. The filing will also be emailed to you when the download completes.
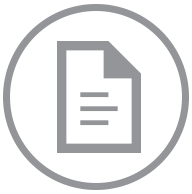
Your document is on its way!
If you do not receive the document in five minutes, contact support at support@docketalarm.com.
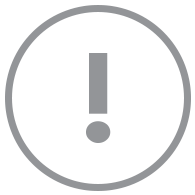
Sealed Document
We are unable to display this document, it may be under a court ordered seal.
If you have proper credentials to access the file, you may proceed directly to the court's system using your government issued username and password.
Access Government Site