`Ž
`Brain Research Reviews 29 1999 169–195
`
`Full-length review
`EEG alpha and theta oscillations reflect cognitive and memory
`performance: a review and analysis
`Wolfgang Klimesch )
`Department of Physiological Psychology, Institute of Psychology, UniÕersity of Salzburg, Hellbrunnerstr. 34, A-5020 Salzburg, Austria
`Accepted 24 November 1998
`
`Abstract
`
`Evidence is presented that EEG oscillations in the alpha and theta band reflect cognitive and memory performance in particular. Good
`Ž .
`Ž .
`performance is related to two types of EEG phenomena i a tonic increase in alpha but a decrease in theta power, and ii a large phasic
`Ž
`.
`event-related decrease in alpha but increase in theta, depending on the type of memory demands. Because alpha frequency shows large
`interindividual differences which are related to age and memory performance, this double dissociation between alpha vs. theta and tonic
`vs. phasic changes can be observed only if fixed frequency bands are abandoned. It is suggested to adjust the frequency windows of alpha
`and theta for each subject by using individual alpha frequency as an anchor point. Based on this procedure, a consistent interpretation of a
`Ž
`variety of findings is made possible. As an example, in a similar way as brain volume does, upper alpha power increases but theta power
`.
`decreases from early childhood to adulthood, whereas the opposite holds true for the late part of the lifespan. Alpha power is lowered
`and theta power enhanced in subjects with a variety of different neurological disorders. Furthermore, after sustained wakefulness and
`during the transition from waking to sleeping when the ability to respond to external stimuli ceases, upper alpha power decreases, whereas
`Ž
`.
`theta increases. Event-related changes indicate that the extent of upper alpha desynchronization is positively correlated with semantic
`long-term memory performance, whereas theta synchronization is positively correlated with the ability to encode new information. The
`reviewed findings are interpreted on the basis of brain oscillations. It is suggested that the encoding of new information is reflected by
`Ž
`.
`theta oscillations in hippocampo-cortical feedback loops, whereas search and retrieval processes in semantic long-term memory are
`reflected by upper alpha oscillations in thalamo-cortical feedback loops. q 1999 Elsevier Science B.V. All rights reserved.
`
`Keywords: EEG; ERD; Alpha; Theta; Oscillation; Memory; Hippocampus; Thalamus
`
`Contents
`1. Introduction . . . . . . . . . . . . . . . . . . . . . . . . . . . . . . . . . . . . . . . . . . . . . . . . . . . . . . . . . . . . . . . . . . . . . . . .
`1.1. Cognitive and memory performance: introductory remarks . . . . . . . . . . . . . . . . . . . . . . . . . . . . . . . . . . . . . . . . . . . .
`1.2. Alpha and theta: some basic aspects . . . . . . . . . . . . . . . . . . . . . . . . . . . . . . . . . . . . . . . . . . . . . . . . . . . . . . . .
`1.3. Definition of frequency bands. . . . . . . . . . . . . . . . . . . . . . . . . . . . . . . . . . . . . . . . . . . . . . . . . . . . . . . . . . . .
`1.4. Oscillatory components in the EEG. . . . . . . . . . . . . . . . . . . . . . . . . . . . . . . . . . . . . . . . . . . . . . . . . . . . . . . . .
`2. Tonic changes and differences in the alpha and theta frequency range . . . . . . . . . . . . . . . . . . . . . . . . . . . . . . . . . . . . . . . . .
`2.1. Age related changes and performance related differences in alpha frequency . . . . . . . . . . . . . . . . . . . . . . . . . . . . . . . . . . .
`2.2. Age related changes in alpha and theta power . . . . . . . . . . . . . . . . . . . . . . . . . . . . . . . . . . . . . . . . . . . . . . . . . . .
`2.3. Age related changes in alpha and theta reactivity. . . . . . . . . . . . . . . . . . . . . . . . . . . . . . . . . . . . . . . . . . . . . . . . . .
`2.4. Changes in alpha and theta power during the wake–sleep cycle . . . . . . . . . . . . . . . . . . . . . . . . . . . . . . . . . . . . . . . . . .
`2.5. Alpha power in congenital blindness . . . . . . . . . . . . . . . . . . . . . . . . . . . . . . . . . . . . . . . . . . . . . . . . . . . . . . . .
`3. Interim discussion. . . . . . . . . . . . . . . . . . . . . . . . . . . . . . . . . . . . . . . . . . . . . . . . . . . . . . . . . . . . . . . . . . . . .
`4. Event-related phasic changes in the alpha and theta band . . . . . . . . . . . . . . . . . . . . . . . . . . . . . . . . . . . . . . . . . . . . . . .
`Ž
`.
`4.1. Desynchronization in the lower alpha band reflects attention . . . . . . . . . . . . . . . . . . . . . . . . . . . . . . . . . . . . . . . . . . .
`4.2. Desynchronization in the upper alpha band reflects semantic memory performance . . . . . . . . . . . . . . . . . . . . . . . . . . . . . . .
`4.3. Synchronization in the theta band reflects episodic memory and the encoding of new information . . . . . . . . . . . . . . . . . . . . . . .
`4.4. The relationship between desynchronization, synchronization and absolute power . . . . . . . . . . . . . . . . . . . . . . . . . . . . . . . .
`
`170
`170
`171
`172
`174
`174
`175
`176
`178
`178
`181
`181
`182
`183
`185
`187
`189
`
`) Fax: q43-662-8044-5126; e-mail: wolfgang.klimesch@sbg.ac.at
`
`0165-0173r99r$ - see front matter q 1999 Elsevier Science B.V. All rights reserved.
`Ž
`.
`PII: S0165-0173 98 00056-3
`
`Wave Neuroscience EX2002
`PeakLogic v. Wave Neuroscience
`IPR2022-01550
`
`
`
`170
`
`W. KlimeschrBrain Research ReÕiews 29 1999 169–195
`)
`(
`
`5. General conclusions and physiological considerations. . . . . . . . . . . . . . . . . . . . . . . . . . . . . . . . . . . . . . . . . . . . . . . . . .
`
`Acknowledgements. . . . . . . . . . . . . . . . . . . . . . . . . . . . . . . . . . . . . . . . . . . . . . . . . . . . . . . . . . . . . . . . . . . . . .
`
`References . . . . . . . . . . . . . . . . . . . . . . . . . . . . . . . . . . . . . . . . . . . . . . . . . . . . . . . . . . . . . . . . . . . . . . . . . .
`
`189
`
`191
`
`191
`
`1. Introduction
`
`In a physiological sense, EEG power reflects the num-
`ber of neurons that discharge synchronously. Because brain
`volume and the thickness of the cortical layer is positively
`w
`x.
`Ž
`correlated with intelligence
`e.g., Refs. 12,165
`it
`is
`tempting to assume that EEG power too, is a measure that
`reflects the capacity or performance of cortical information
`processing. Although it will be argued that
`this is in
`principle the case,
`it must be emphasized that power
`measurements are strongly affected by a variety of unspe-
`cific factors such as the thickness of the skull or the
`volume of cerebrospinal fluid, by methodological and tech-
`Ž
`nical factors
`such as interelectrode distance or type of
`.
`montage but also by more specific factors such as age,
`arousal and the type of cognitive demands during actual
`task performance.
`It is the purpose of the present article to show that EEG
`power is indeed related to cognitive and memory perfor-
`mance, but in a complex and partly non-linear way. Within
`the alpha frequency range EEG power is positively related
`to cognitive performance and brain maturity, whereas the
`opposite holds true for the theta frequency range. Alpha
`and theta reactivity as well as event-related changes in
`alpha and theta band power show yet another pattern of
`results. During actual task demands the extent of alpha
`power suppression is positively correlated with cognitive
`Ž
`.
`performance
`and memory performance in particular
`whereas again the opposite holds true for the theta band.
`Here, the extent of theta synchronization is related to good
`performance. The review which focuses on the theta and
`alpha frequency range considers first
`tonic changes in
`Ž
`.
`power
`such as age related differences in the EEG and
`then phasic or event-related changes.
`
`1.1. CognitiÕe and memory performance: introductory re-
`marks
`
`Two basic aspects of memory processes will be distin-
`w
`x
`guished 66 . The first refers to processes of the working
`Ž
`.
`memory system WMS , the second to that of the long-term
`Ž
`.
`memory system LTMS . Probably any cognitive process
`depends on the resources of both systems. As an example,
`let us consider an every day cognitive process such as
`recognizing a familiar object. The basic idea here is that
`after a sensory code is established, semantic information in
`Ž
`.
`long-term memory LTM is accessed which is used to
`identify the perceived object. If the matching process
`
`yields a positive result, the object is recognized which in
`Ž
`.
`turn leads to the creation of a short-term memory STM
`code. In this case, bottom up pathways are activated which
`are similar or identical to those which would serve to
`retrieve information from LTM. This classical explanation
`of encoding still reflects the current view, which was
`w
`x
`originally stated by Shiffrin and Geisler 137 : ‘‘The pro-
`cess of encoding is essentially one of recognition:
`the
`appropriate image or feature is contacted in LTM and then
`Ž
`.
`Ž
`.
`placed i.e., copied in STM’’ p. 55 . Complex cognitive
`processes such as speaking and thinking may also be
`described in terms of a close interaction between the WMS
`and LTMS. The basic difference to the foregoing example
`is that a sensory code is lacking and that a code is
`generated in STM which in the case of speaking represents
`a ‘plan’ of what to say. The codes generated in STM
`trigger search processes in LTM to retrieve the relevant
`knowledge about the appropriate semantic, syntactic and
`articulatory information. This latter idea is similar to Bad-
`deley’s concept of working memory, which comprises an
`attentional controller, the central executive and subsidiary
`w
`x
`slave systems 3,4 .
`In the WMS, encoding has two different meanings, one
`refers to episodic, the other to sensory-semantic informa-
`Ž
`tion. The encoding of sensory information as a process of
`.
`recognition always aims at the semantic understanding of
`perceived information which is first processed in the
`LTMS. Because of this close relation between sensory and
`semantic encoding we will use the term sensory-semantic
`code. The creation of a new code comprises episodic
`w
`x.
`Ž
`information, which according to Tulving e.g., 158 , is
`that type of contextual information which keeps an individ-
`ual autobiographically oriented within space and time.
`Because time changes the autobiographical context perma-
`nently, there is a permanent and vital need to update and
`store episodic information. Thus, the formation of episodic
`memory traces is one of the most important tasks of the
`WMS.
`Cognitive performance is closely related and linked to
`the performance of the WMS and LTMS. As an example,
`most intelligence tests comprise subtests measuring mem-
`Ž
`.
`ory span an important function of the WMS and tests
`Ž
`such as judging analogies which reflect an important
`.
`aspect of semantic LTMS . The increase in cognitive
`performance from childhood to puberty as well as the
`decrease in performance during the late lifespan is, most
`likely, due or at least closely linked to performance changes
`in the WMS and LTMS.
`
`
`
`W. KlimeschrBrain Research ReÕiews 29 1999 169–195
`)
`(
`
`171
`
`to the functional anatomy of memory,
`With respect
`there is good evidence that brain structures that lie in the
`Ž
`medial temporal lobe comprising the hippocampal forma-
`.
`tion and prefrontal cortex support various functions of the
`w
`x
`Ž
`WMS cf. the reviews in Refs. 102,140,141 and findings
`about the contribution of the hippocampal region for the
`generation of event-related potentials in the human scalp
`w
`x.
`EEG during novelty detection 85,86 . Studies focusing on
`the ontogeny of human memory indicate that
`the hip-
`pocampus matures relatively early in postnatal life, whereas
`the prefrontal cortex which is important for the develop-
`Ž
`ment of an increased memory span matures much later cf.
`w
`x
`.
`Refs. 109,57 for reviews . Although many aspects of
`Ž
`memory develop early in childhood up to an age of about
`.
`2 or 3 years it is not yet known when memory is fully
`matured. As will be discussed in Section 5, there is good
`Ž
`evidence that a complex structure of feedbackloops or
`w
`x.
`connecting the hippocampus with
`‘reentrant loops’; 37
`different cortical regions and the prefrontal cortex in par-
`ticular may provide the anatomical basis for the WMS. It
`appears likely that these feedbackloops develop and be-
`come increasingly differentiated with increasing age over
`the entire time span of childhood and possibly early adult-
`hood. The increase in EEG frequency during that
`life
`Ž
`.
`period may besides other factors reflect this process of
`brain maturation. The basic assumption is that the better
`these feedbackloops become integrated and interconnected
`with other brain areas, the faster the frequency of EEG
`oscillations will be.
`
`1.2. Alpha and theta: some basic aspects
`
`Ž
`Alpha is with the exception of irregular activity in the
`.
`delta range and below the dominant frequency in the
`human scalp EEG of adults. It is manifested by a ‘peak’ in
`Ž
`.
`spectral analysis cf. Fig. 1 and reflects rhythmic ‘alpha
`w
`x.
`Ž
`waves’ which are known since Berger e.g., Ref. 10 . The
`fact that alpha clearly is an oscillatory component of the
`human EEG has led to a recent ‘renaissance’ in the interest
`w
`x
`of EEG alpha activity 5,8,7,9 .
`Frequency and power are closely interrelated measures.
`Usually, alpha frequency is defined in terms of peak or
`gravity frequency within the traditional alpha frequency
`Ž
`.
`range f 1 to f 2 of about 7.5–12.5 Hz. Peak frequency is
`that spectral component within f 1 to f 2 which shows the
`Ž
`.
`largest power estimate cf. Fig. 1A . Alpha frequency can
`Ž
`.
`also be calculated in terms of gravity or ‘mean’
`fre-
`quency which is the weighted sum of spectral estimates,
`divided by alpha power: Ý a f =f r Ý a f
`Ž
`Ž Ž
`.
`..
`Ž
`Ž
`..
`. Power
`Ž
`.
`spectral estimates at frequency f are denoted a f . The
`index of summation is in the range of f 1 to f 2. Particu-
`Ž
`larly if there are multiple peaks in the alpha range for a
`w
`x.
`classification see e.g., Ref. 39 , gravity frequency appears
`the more adequate estimate of alpha frequency.
`As is well known from animal research, unlike alpha in
`the human scalp EEG, theta is the dominant rhythm in the
`
`.
`Ž
`Fig. 1. Individual alpha frequency IAF varies to a large extent even in a
`sample of age matched subjects as the three power spectra indicate.
`Depending on their memory performance and other factors, IAF lies in
`Ž
`.
`the range of about 9.5–11.5 Hz for young healthy adults. A If power
`spectra are calculated separately for a resting period in which subjects are
`Ž
`.
`in a state of alert wakefulness dotted line
`and during actual
`task
`Ž
`.
`performance e.g., memorizing visually presented words , alpha power
`Ž
`.
`becomes suppressed, but theta power increases bold line . That fre-
`quency where the two spectra intersect marks the transition frequency
`
`Ž .TF between the theta and alpha band. Experiments from our laboratory
`have shown that TF lies almost 4 Hz below IAF and that the lower alpha
`Ž
`.
`band which can be further divided in two subbands is somewhat wider
`Ž .
`than the upper alpha band. B Alpha power of a subject with a very slow
`alpha rhythm will fall outside the fixed frequency window of the tradi-
`Ž
`.
`tional alpha band of about 7.5–12.5 Hz. As an example, if IAF in A is
`Ž .
`Ž .
`10 Hz and in B only 8 Hz, the lower alpha band in B will already
`Ž
`.
`comprise that frequency range which is the theta band in A , whereas the
`Ž .
`upper alpha band in B will fall in the frequency range of the lower
`Ž
`.
`alpha band in A . Similar problems arise, if IAF lies already at the upper
`Ž .
`frequency limit of the alpha band. C For a subject with a very fast IAF
`of about 11.5 Hz or more, almost the entire frequency range of the
`Ž
`.
`traditional alpha band 7.5–12.5 Hz would actually fall in the range of
`the lower alpha band of that subject. It is therefore of crucial importance
`to adjust frequency bands individually for each subject, by using IAF as
`Ž
`.
`the cut off point between the lower and upper alpha band see text .
`
`
`
`172
`
`W. KlimeschrBrain Research ReÕiews 29 1999 169–195
`)
`(
`
`hippocampus of lower mammals. Its frequency ranges
`w
`x.
`Ž
`from about 3 to 12 Hz e.g., Ref. 95 and, thus, shows a
`much wider frequency range than in humans where theta
`lies between about 4 to 7.5 Hz. Its wide frequency range
`and large power make it easy to observe frequency and
`power changes in animals. This is in sharp contrast to the
`human scalp EEG, where—without the help of sophisti-
`cated methods—changes in theta frequency are very diffi-
`cult or almost impossible to detect. The question, thus, is
`whether there is a physiological criterion that allows us to
`decide which frequency marks the transition between alpha
`and theta oscillations.
`Alpha and theta respond in different and opposite ways.
`The crucial finding is that with increasing task demands
`Ž
`theta synchronizes, whereas alpha desynchronizes cf. the
`.
`bold in relation to dotted line in Fig. 1A . This fact is
`documented in reviews on event-related desynchronization
`. w
`x
`Ž
`ERD 122,67,68 , as well as by a variety of studies using
`w
`Ž
`other experimental approaches
`e.g., Ref. 45,46,95,99,
`x.
`131,133,129 . If EEG power in a resting condition is
`compared with a test condition, alpha power decreases
`Ž
`.
`Ž
`.
`desynchronizes and theta power increases synchronizes .
`Classical findings demonstrate that the decrease in alpha
`Ž
`.
`power suppression of the alpha rhythm can be observed
`primarily when subjects close their eyes. More recent
`evidence, however, suggests that attentional and semantic
`memory demands are powerful factors which lead to a
`selective suppression of alpha in different ‘subbands’ and
`Ž
`that the well described effects of visual stimulation e.g.,
`.
`just a special class of
`eyes open vs. closed represent
`Ž
`sensory-semantic task demands
`see Section 4 and Refs.
`w
`x.
`72,75,78–81 . As already emphasized, encoding of sen-
`sory information always aims to extract the meaning of the
`perceived information which is stored in semantic LTM.
`Thus, there is a close relation between sensory and seman-
`tic encoding.
`As Fig. 1 illustrates, that frequency in the power spectra
`which marks the transition from theta synchronization to
`alpha desynchronization may be considered the individual
`Ž
`.
`transition frequency TF between the alpha and theta band
`for each subject. When using this method to estimate TF,
`we have found that TF shows a large interindividual
`Ž
`.
`variability ranging from about 4 to 7 Hz which is signifi-
`w
`x
`cantly correlated with alpha peak frequency 76 . Prelimi-
`nary evidence for a covariation between theta and alpha
`w
`x
`frequency was already found by Klimesch et al. 75 and is
`w
`x
`further documented by Doppelmayr et al. 34 . These
`Ž
`.
`findings indicate that theta frequency as measured by TF
`varies as a function of alpha frequency and suggest to use
`alpha frequency as a common reference point for adjusting
`different frequency bands not only for the alpha, but theta
`range as well. For estimating theta power, the individual
`determination of frequency bands may even be more im-
`portant because otherwise the effects of theta synchroniza-
`tion are masked by alpha desynchronization particularly in
`Ž
`.
`the range of TF cf. Fig. 1B,C .
`
`1.3. Definition of frequency bands
`
`Because alpha frequency varies to a large extent as a
`function of age, neurological diseases, memory perfor-
`w
`x
`Ž
`.
`mance see Section 2.1 below , brain volume 115,114
`w
`x
`and task demands 73 , the use of fixed frequency bands
`does not seem justified. As an example, an elderly subject
`with bad memory performance may show a peak fre-
`w
`x
`quency of 7 Hz or lower 16 . When strictly applying the
`rule that alpha peak frequency is that spectral component
`within f 1s7.5 and f 2s12. 5 Hz which shows maximal
`power, we would arrive at the conclusion that the obtained
`frequency indicates theta instead of alpha frequency. For-
`tunately, as discussed in the previous section, there is a
`physiological criterion which allows us to answer this
`question. If EEG power around 7 Hz would desynchronize
`Ž
`during a test—as compared to a resting condition cf. Fig.
`.1A —we still would accept that a peak frequency of 7 Hz
`indicates alpha but not theta frequency.
`This example documents the necessity to define the
`Ž
`alpha band individually for each subject as that range
`f 1
`.
`to f 2 ‘around’ the individual dominant EEG frequency
`Ž
`.
`above the lower delta range that desynchronizes during
`task demands. In order to avoid confusions with traditional
`measures, we use the term individual alpha frequency
`Ž
`.
`Ž
`.
`IAF to denote the individual dominant peak or gravity
`Ž
`.
`EEG frequency in the range of
`f 1 to f 2 of a single
`subject. The crucial point, of course, is the exact location
`and individual definition of the frequency limits f 1 and
`f 2. For f 1, TF is a good estimate, but for f 2 an obvious
`physiological criterion is lacking. An indirect way to solve
`this problem is to define the frequency limits of the lower
`alpha band by f 1 and IAF and to assign the ‘remaining’
`w
`Ž
`part of the alpha frequency range which equals alpha
`x
`w
`x.
`frequency window y IAFyf 1
`to the upper band. Re-
`sults from our laboratory indicate that the lower alpha
`band has a width of about 3.5–4 Hz. Accordingly, the
`Ž
`.
`upper alpha band the frequency range above IAF is a
`rather narrow band of 1 or 1.5 Hz, if it is assumed that the
`alpha frequency window has a width of about 5 Hz.
`Ž
`Experimental findings discussed in detail in Section 4
`.
`below indicate that the upper alpha band—defined as a
`band of 2 Hz above IAF—responds selectively to semantic
`LTM demands and behaves in a completely different and
`Ž
`sometimes opposite way as the lower alpha band see also
`w
`x.
`the review in Ref. 121 . Furthermore and most impor-
`Ž
`tantly, it was found that the lower alpha band a band of 4
`.
`Hz below IAF reflects different types of attentional de-
`mands. Thus, it was broken down into two subbands of 2
`Ž
`Hz each which are termed lower-1 and lower-2 alpha see
`.
`Fig. 1 and Section 4 .
`In summarizing, when using IAF as an anchor point, it
`Ž
`proved useful
`to distinguish three alpha bands with a
`.
`Ž
`.
`width of 2 Hz each , two lower alpha bands below IAF
`Ž
`.
`and one upper alpha band above IAF . The theta band is
`defined as the frequency band of 2 Hz which falls below
`
`
`
`W. KlimeschrBrain Research ReÕiews 29 1999 169–195
`)
`(
`
`173
`
`TF. As for the upper frequency limit of the upper alpha
`band, there are no clear criteria for the lower frequency
`limit of the theta band. In any case, however, it is impor-
`tant to emphasize that the use of narrow frequency bands
`reduces the danger that frequency specific effects go unde-
`tected or cancel each other. Thus, broad band analyses
`must be interpreted with great caution. The implication is
`that an unbiased estimate of alpha and theta power can be
`obtained only, if the traditional fixed band analyses are
`abandoned and if narrow frequency bands are adjusted to
`the individual alpha frequency of each subject. However,
`the vast majority of studies use broad, instead of narrow
`Ž
`.
`alpha bands cf. Table 1 which in addition are not ad-
`justed individually.
`The suggested definition of frequency bands is based on
`Ž
`.
`physiological criteria such as TF and IAF and on the
`Ž
`functional significance of narrow frequency bands
`see
`.
`Section 4 . An alternative way to define EEG frequency
`bands is to analyze the covariance of spectral estimates by
`multivariate statistical methods such as factor analysis. The
`crucial question here is whether the activities in different
`frequency bands vary independently. With respect to the
`traditional theta and alpha frequency range, results from
`
`factor analyses show at least three independent factors, one
`for theta, one for the lower alpha band and another for the
`w
`x
`Ž
`upper alpha band see e.g., Ref. 106 and the summary in
`w
`x.
`Ref. 96 . However, the frequency limits vary consider-
`Ž
`ably between studies. As an example, Wieneke reported
`w
`x.
`found a factor covering the frequency range
`in Ref. 96
`of 6–9 Hz, which was termed ‘theta’ whereas within that
`w
`x
`same frequency range, Mecklinger et al. 106 extracted
`one component which they classified as ‘lower alpha’.
`Divergent results from factor analyses are due to the type
`Ž
`of power measurements
`spectral estimates may be ex-
`.
`pressed in terms of relative or absolute power , type of
`w
`x
`Ž
`referential , bipolar, Lapla-
`derivation e.g., monopolar
`.
`Ž
`cian , electrode location, task type resting condition with
`.
`eyes open or closed or performance of some task , the
`selected sample of subjects, and finally the method used to
`extract and rotate factors. It also should be emphasized that
`factor analyses are usually performed on the basis of a
`correlation matrix which was obtained by correlating spec-
`tral estimates over a sample of n subjects. Done in this
`way,
`the extracted factors represent average frequency
`ranges of that particular sample. However, factor analysis
`could also be used for an individual definition of fre-
`
`Der.
`M
`M
`M
`M
`
`Table 1
`ŽU .
`.
`Ž
`frequency
`EEG alpha activity: frequency limits and peak a or gravity
`Ref. no.rauthor
`Broad band
`Subbands
`Age
`a-frequency
`N
`w x1 Alloway et al.
`8–12
`–
`10
`29–62
`–
`w x2 Anokhin and Vogel
`8–13
`–
`101
`20–45
`–
`
`w x11 Besthorn et al.
`–
`7.5–9.5; 9.5–12.5
`92
`59–78
`–
`
`w x14 Boiten et al.
`8–13
`–
`8
`22–29
`–
`
`w x16 Brenner et al.
`–
`8.0–9.9; 10–12.9
`119
`51–89
`6–14,r
`
`w x17 Breslau et al.
`8.2–12.9
`–
`33
`18–78
`–
`
`w x24 Chiaramonti et al.
`8–11.5
`–
`55
`51–81
`–
`
`w x27 Coben et al.
`U
`8–13
`–
`127
`64–83
`6.9–9.3
`
`w x36 Duffy et al.
`8–11.75
`–
`63
`30–80
`7.5–13a,r
`
`w x44 Gevins et al.
`8–13
`–
`55
`–
`–
`
`w x55 Hartikainen et al.
`7.6–13.9
`–
`52
`33–78
`9.6–10.1a
`
`w x61 Jausovec
`7.5–13
`–
`60
`18–19
`–
`
`w x62 John et al.
`7.5–12.5
`–
`648
`1–21
`–
`
`w x65 Kaufman et al.
`8–12
`–
`3
`–
`–
`
`w x87 Kononen and Partanen
`¨ ¨
`7.6–13.9
`–
`54
`23–80
`–
`
`w x89 Krause et al.
`–
`8–10; 10–12
`10
`23–41
`–
`
`w x99 Marciani et al.
`8–12.5
`–
`60
`19–89
`–
`
`w x117 Obrist et al.
`–
`–
`57
`65–84
`7.5–9.2a
`
`w x124 Pfurtscheller
`–
`1 or 2 Hz bands
`M, B
`§
`§
`–
`
`w x132 Salenius et al.
`7–14 Hz
`–
`MEG
`13
`20–49
`9.3–11a,r
`
`w x134 Schmid et al.
`7.4–12.5
`–
`B
`536
`5–11
`–
`
`w x139 Somsen et al.
`7.5–12.5
`–
`M
`142
`5–12
`4.5–10a
`
`w x146 Sterman et al.
`–
`2 Hz bands 5–15
`M
`26
`25–39
`–
`
`w x153 Szelies et al.
`8–13
`–
`M
`58
`53–78
`–
`
`w x156 Torres et al.
`9.4$,S.D.s1
`–
`–
`M
`182
`48–88
`
`w x157 Torsvall and Akerstedt
`8–11.9
`–
`B
`11
`27–58
`–
`
`w x162 Wada et al.
`7.5–12.5
`–
`M
`264
`3–26
`–
`
`w x164 Wieneke et al.
`9.9a,S.D.s1
`3 db below peak
`M
`110
`18–50
`
`w x166 Williamson et al
`–
`8–13
`MEG
`§
`§
`–
`Der.sDerivation; Msmonopolar; Bsbipolar, CsCommon average reference; Nssample size; §, Reviewed data; r, range for lowest and highest
`frequency; $, Dominant frequency, defined by period of alpha wave.
`
`M
`M
`B
`M
`M
`B
`M
`B
`MEG
`B
`M
`C
`
`
`
`174
`
`W. KlimeschrBrain Research ReÕiews 29 1999 169–195
`)
`(
`
`quency bands, if the EEG of a single subjects is used and
`if spectral estimates are correlated over a series of n trials
`Ž
`.
`epochs .
`Even if frequency bands are defined individually for
`each subject, it must be emphasized that EEG frequencies
`vary between recording sites. As an example, it is well
`known that alpha waves occur primarily during wakeful-
`ness over the posterior regions of the head and can be best
`seen with eyes closed and under conditions of physical
`w
`x
`relaxation and mental inactivity 110 . The frequency of
`alpha waves is faster at posterior and slower at anterior
`recording sites. It would,
`thus, be desirable to adjust
`frequency bands not only individually for each subject but
`also for each recording site. For practical reasons, this has
`not yet been done.
`
`1.4. Oscillatory components in the EEG
`
`is
`In an empirical sense, an oscillatory component
`defined by the presence of a rhythmic activity in the EEG
`which is manifested by a ‘peak’ in spectral analysis. In
`contrast to theta, alpha as the dominant rhythmic activity,
`characterized by sinusoidal wave forms, clearly meets this
`definition. In the human EEG of young healthy adults,
`there are at least two other oscillatory components, the mu
`Ž
`rhythm and the third rhythm. The mu rhythm mu stands
`.
`for motor has an arch-shaped wave morphology, appears
`Ž
`over the motor area and becomes suppressed desynchro-
`.
`Ž
`nized during motor related task demands see the exten-
`w
`x.
`sive work of Pfurtscheller et al., e.g., Ref. 125 . Other
`terms are ‘arcade’, ‘comb’ or ‘wicket’ rhythm, ‘central’,
`Ž
`‘rolandic’ and ‘somatosensory’ alpha cf. the review in
`w
`x
`.
`Ref. 110 p. 137f . The third rhythm which is not de-
`Ž
`tectable in the scalp EEG but e.g., by the use of epidural
`w
`x.
`electrodes or magnetoencephalography MEG is indepen-
`Ž
`.
`dent from the posterior alpha and mu rhythm and appears
`w
`x
`over the midtemporal region 110 . In emphasizing the fact
`Ž
`.
`in the MEG this rhythm is best seen over the
`that
`w
`x
`auditory cortex in the temporal lobe, Hari 51 uses the
`Ž
`.
`term ‘tau’ rhythm tau stands for temporal . There is some
`evidence that the tau rhythm becomes suppressed during
`Ž
`acoustic but not visual stimulation cf. the review in Hari
`w
`x.
`et al. 52 . Because the focus of this review is on theta and
`alpha activity with respect to cognitive performance and
`memory, the mu and third rhythm will not be considered.
`Despite the fact that theta does not meet the criteria for
`an oscillatory component
`in the EEG,
`it may still be
`argued that activity within the individually defined theta
`band reflects oscillatory processes. In a theoretical sense,
`the EEG can be conceived of a linear superposition of a set
`Ž
`.
`of different sine waves oscillatory components . In addi-
`tion, there are more specific arguments which are based on
`empirical evidence:
`1. With the help of sophisticated methods, theta peaks can
`be found in the human scalp EEG of young healthy
`w
`x
`adults 45 .
`
`Table 2
`.
`Ž
`Double dissociation between tonic and phasic event-related changes in
`alpha and theta power with respect to cognitive performance
`Increasing performance
`Decreasing performance
`Theta power Alpha power Theta power Alpha power
`Tonic change Decreases
`Increases
`Increases
`Decreases
`Phasic change Increases
`Decreases
`Decreases
`Increases
`
`Tonic changes are discussed in Section 2, phasic changes in Section 4
`below.
`A phasic change is measured as an increase or decrease in band power
`Ž
`during task performance as compared to a reference or resting period cf.
`.
`Fig. 7 .
`
`.
`Ž
`2. Theta frequency as measured by TF covaries with
`w
`x.
`Ž
`alpha frequency as measured by IAF 34,76 .
`3. Theta and alpha band power are related to each other,
`Ž
`although in a reciprocal or ‘opposite’ way see Sections
`.
`2–4 below and Table 2 ,
`theta clearly is an
`4. Animal research has shown that
`oscillatory component of the hippocampal EEG which
`Ž
`is related to memory processes see e.g., the review by
`w
`x
`.
`Miller 107 and Section 5 below ,
`5. Research from our laboratory indicates that theta band
`power increases in response to memory demands just as
`Ž
`hippocampal theta in animals does
`see Sections 4.3
`.
`and 5 below .
`Thus, the concepts of desynchronization and synchro-
`Ž
`.
`nization will also be used for the individually defined
`theta band. In a similar way, these concepts will be applied
`to the different subbands of alpha. Visual inspection of
`‘alpha waves’ in the EEG may invite the misleading
`interpretation that there is only a single rhythm which may
`just vary in frequency. As the results from factor analyses
`have shown, there are at least two independent components
`of alpha activity which must be distinguished. Based on
`physiological and experimental evidence, many authors
`meanwhile assume that there is an entire population of
`w
`x.
`Ž
`different alpha rhythms e.g., Refs. 6,166,167 . Thus, it
`seems quite obvious to assume that during desynchroniza-
`tion different alpha rhythms in different subbands start to
`Ž
`oscillate with different frequencies
`for more details see
`.
`Section 5 .
`
`2. Tonic changes and differences in the alpha and theta
`frequency range
`
`The type of EEG changes or differences which are
`discussed in the following sections my be termed ‘tonic’ in
`Ž
`order to contrast them from ‘phasic’ changes. Phasic or
`.
`event-related changes in the EEG are more or less under
`volitional control and occur at a rapid rate, whereas tonic
`Ž
`.
`changes are not or less under volitional control and occur
`at a much slower rate. Phasic changes in the EEG are task
`andror stimulus related. Tonic changes, on the other hand,
`
`
`
`W. KlimeschrBrain Research ReÕiews 29 1999 169–195
`)
`(
`
`175
`
`occur over the life cycle and in response to circadian
`rhythms, fatigue, distress, neurological disorders, etc.
`
`2.1. Age related changes and performance related differ-
`ences i
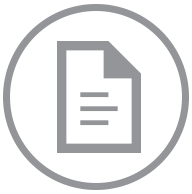
Accessing this document will incur an additional charge of $.
After purchase, you can access this document again without charge.
Accept $ ChargeStill Working On It
This document is taking longer than usual to download. This can happen if we need to contact the court directly to obtain the document and their servers are running slowly.
Give it another minute or two to complete, and then try the refresh button.
A few More Minutes ... Still Working
It can take up to 5 minutes for us to download a document if the court servers are running slowly.
Thank you for your continued patience.
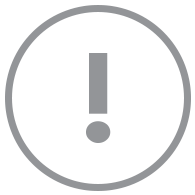
This document could not be displayed.
We could not find this document within its docket. Please go back to the docket page and check the link. If that does not work, go back to the docket and refresh it to pull the newest information.
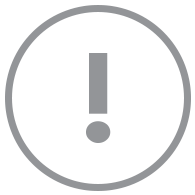
Your account does not support viewing this document.
You need a Paid Account to view this document. Click here to change your account type.
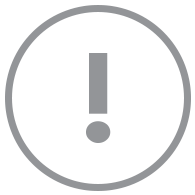
Your account does not support viewing this document.
Set your membership
status to view this document.
With a Docket Alarm membership, you'll
get a whole lot more, including:
- Up-to-date information for this case.
- Email alerts whenever there is an update.
- Full text search for other cases.
- Get email alerts whenever a new case matches your search.
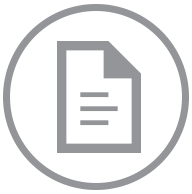
One Moment Please
The filing “” is large (MB) and is being downloaded.
Please refresh this page in a few minutes to see if the filing has been downloaded. The filing will also be emailed to you when the download completes.
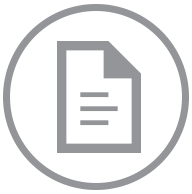
Your document is on its way!
If you do not receive the document in five minutes, contact support at support@docketalarm.com.
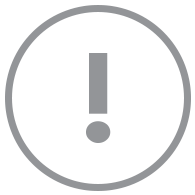
Sealed Document
We are unable to display this document, it may be under a court ordered seal.
If you have proper credentials to access the file, you may proceed directly to the court's system using your government issued username and password.
Access Government Site