`First published September 19, 2006; doi:10.1152/ajpendo.00215.2006.
`
`Are blood flow and lipolysis in subcutaneous adipose tissue influenced by
`contractions in adjacent muscles in humans?
`
`Bente Stallknecht, Flemming Dela, and Jørn Wulff Helge
`Department of Medical Physiology, The Copenhagen Muscle Research Centre,
`The Panum Institute, University of Copenhagen, Copenhagen, Denmark
`
`Submitted 5 May 2006; accepted in final form 11 September 2006
`
`Stallknecht B, Dela F, Helge JW. Are blood flow and lipolysis in
`subcutaneous adipose tissue influenced by contractions in adjacent mus-
`cles in humans? Am J Physiol Endocrinol Metab 292: E394–E399, 2007.
`First published September 19, 2006; doi:10.1152/ajpendo.00215.2006.—
`Aerobic exercise increases whole body adipose tissue lipolysis, but is
`lipolysis higher in subcutaneous adipose tissue (SCAT) adjacent to
`contracting muscles than in SCAT adjacent to resting muscles? Ten
`healthy, overnight-fasted males performed one-legged knee extension
`exercise at 25% of maximal workload (Wmax) for 30 min followed by
`exercise at 55% Wmax for 120 min with the other leg and finally exercised
`at 85% Wmax for 30 min with the first leg. Subjects rested for 30 min
`between exercise periods. Femoral SCAT blood flow was estimated from
`washout of 133Xe, and lipolysis was calculated from femoral SCAT
`interstitial and arterial glycerol concentrations and blood flow. In general,
`blood flow and lipolysis were higher in femoral SCAT adjacent to
`contracting than adjacent to resting muscle (time 15–30 min; blood flow:
`25% Wmax 6.6 ⫾ 1.0 vs. 3.9 ⫾ 0.8 ml䡠100 g⫺1 䡠min⫺1, P ⬍ 0.05; 55%
`Wmax 7.3 ⫾ 0.6 vs. 5.0 ⫾ 0.6 ml䡠100 g⫺1 䡠min⫺1, P ⬍ 0.05; 85% Wmax
`6.6 ⫾ 1.3 vs. 5.9 ⫾ 0.7 ml䡠100 g⫺1 䡠min⫺1, P ⬎ 0.05; lipolysis: 25%
`Wmax 102 ⫾ 19 vs. 55 ⫾ 14 nmol䡠100 g⫺1 䡠min⫺1, P ⫽ 0.06; 55% Wmax
`86 ⫾ 11 vs. 50 ⫾ 20 nmol䡠100 g⫺1 䡠min⫺1, P ⬎ 0.05; 85% Wmax 88 ⫾
`31 vs. ⫺9 ⫾ 25 nmol䡠100 g⫺1 䡠min⫺1, P ⬍ 0.05). In conclusion, blood
`flow and lipolysis are generally higher in SCAT adjacent to contracting
`than adjacent to resting muscle irrespective of exercise intensity. Thus
`specific exercises can induce “spot lipolysis” in adipose tissue.
`
`exercise; spot lipolysis; microdialysis
`
`OBESITY CAN BE DEFINED AS a condition where the amount of
`adipose tissue is increased to such a degree that it has conse-
`quences for the health of the person. In addition to the amount,
`also the distribution of fat is important. Obesity can be dimin-
`ished by increased physical activity and/or reduced caloric
`intake (11, 21) and, at least in the short term, the size of the
`negative caloric balance is the primary factor determining the
`weight loss (22, 28). There is evidence that exercise-induced
`relative loss of fat is higher in visceral and abdominal subcu-
`taneous adipose tissue (SCAT) than in femoral SCAT (23).
`This indicates that regional adipose tissue depots are regulated
`independently, and for many years it has been discussed
`whether specific exercises can reduce local adipose tissue
`depots, i.e., induce a “spot reduction” of adipose tissue, and
`thus modify fat distribution (19, 20).
`A number of studies have examined the spot reduction
`theory, and conclusions have been contradictory (12, 16, 19,
`20). A valid study design to test
`the hypothesis of spot
`reduction is one in which the muscles in one part of the body
`are trained and the muscles in the contralateral side are not, and
`
`the size of the adipose tissue depots adjacent to the trained
`respective sedentary muscles are measured before and after the
`study period. One study using this design (19) found a decrease
`in skinfold thickness of the trained arm, but in another study
`(20) the skinfold thickness decreased significantly in both the
`trained and the sedentary arm during the study period. The
`latter finding is essentially supported by a study in which the
`skinfold thickness of both arms was measured in tennis players
`and control subjects (12). The skinfold thickness did not differ
`between arms in tennis players or control subjects, but skinfold
`thickness of both arms was lower in tennis players compared
`with controls. Krotkiewski et al. (16) had 10 healthy middle-
`aged females exercise one leg for 5 wk while the other leg was
`resting, and ultrasound measurements revealed that the thick-
`ness of the femoral SCAT of the trained leg was significantly
`decreased, whereas the thickness of the SCAT of the sedentary
`leg was unchanged. However, a reduced thickness of the
`subcutaneous tissue layer could be because of a training-
`induced enlargement of the underlying muscle, which could
`result in a compression of a possibly unchanged amount of
`SCAT. To clarify this, Krotkiewski et al. took two biopsies of
`the SCAT from each thigh and found that fat cell weight
`decreased nonsignificantly in both biopsies from the trained leg
`{⫺7% [0.60 ⫾ (SE) 0.07 to 0.56 ⫾ 0.05 g] and ⫺26%
`(0.42 ⫾ 0.07 to 0.31 ⫾ 0.08 g)} and increased nonsignifi-
`cantly in both biopsies from the sedentary leg [⫹11% (0.54 ⫾
`0.03 to 0.60 ⫾ 0.03 g) and ⫹7% (0.46 ⫾ 0.08 to 0.49 ⫾ 0.08
`g)]. Based on the biopsy data, Krotkiewski et al. turned down
`the spot reduction hypothesis, but it may be speculated that
`their nonsignificant changes in fat cell weight could be because
`of a type 2 statistical error.
`In the present study, we evaluated one component in the spot
`reduction hypothesis, namely if spot lipolysis occurs in SCAT
`overlying contracting skeletal muscle. Our subjects performed
`acute one-legged knee extension exercise, and, by use of 133Xe
`washout and microdialysis techniques, we estimated blood
`flow and lipolysis in femoral SCAT adjacent to contracting and
`resting skeletal muscle.
`
`MATERIALS AND METHODS
`
`Subjects. Ten healthy, moderately active, overnight-fasted males
`[age: 26 ⫾ 2 (SE) yr, weight: 82 ⫾ 3 kg, height: 183 ⫾ 2 cm, BMI:
`24.5 ⫾ 0.8 kg/m2, V˙ O2 max: 49⫾ 2 ml 䡠 kg⫺1 䡠 min⫺1] gave their
`written consent according to the declaration of Helsinki II to partic-
`ipate in the study, which was approved by the Ethics Committee for
`Medical Research in Copenhagen (KF 11– 055/03).
`
`Address for reprint requests and other correspondence: B. Stallknecht, Dept.
`of Medical Physiology, The Panum Institute, Blegdamsvej 3, DK-2200 Copen-
`hagen N, Denmark (e-mail: B.Stallknecht@mfi.ku.dk).
`
`The costs of publication of this article were defrayed in part by the payment
`of page charges. The article must therefore be hereby marked “advertisement”
`in accordance with 18 U.S.C. Section 1734 solely to indicate this fact.
`
`E394
`
`0193-1849/07 $8.00 Copyright © 2007 the American Physiological Society
`Downloaded from journals.physiology.org/journal/ajpendo (038.099.035.066) on November 24, 2021.
`
`http://www.ajpendo.org
`
`LUMENIS EX1084
`Page 1
`
`
`
`SPOT LIPOLYSIS
`
`E395
`
`Protocol. Before the experiment, subjects were accustomed to
`exercise on the knee extension ergometer, and maximal work capacity
`(Wmax) was determined for each leg as described by Andersen et al.
`(1). At least 5 days before the experimental day, subjects performed a
`V˙ O2 peak test on a bicycle ergometer using a standard progressive
`exercise test. Subjects were asked to refrain from vigorous physical
`activity for 2 days before the experiment. After a 12-h fast and
`abstinence from coffee, tea, alcohol, and tobacco, the subjects arrived
`at the laboratory at 8:00 A.M. During the experiments, subjects wore
`shorts and t-shirt, and the room temperature was 23 ⫾ 1°C. A catheter
`(Arterial Cannula with FloSwitch; Becton-Dickinson) was inserted in
`the brachial or radial artery for blood sampling. Microdialysis cathe-
`ters were inserted, and 133Xe was injected in SCAT of both thighs (see
`below). After a 90-min equilibration period, the experiment was
`initiated.
`The experiment consisted of a 15-min resting period and three
`consecutive periods of one-legged knee extension exercise (Fig. 1).
`First subjects exercised with one leg for 30 min at 25% of Wmax (13 ⫾
`1 watts, heart rate 81 ⫾ 3 beats/min). After a 30-min rest, subjects
`exercised with the other leg for 120 min at 55% of Wmax (26 ⫾ 1
`watts, heart rate 84 ⫾ 4 beats/min), and after another 30-min rest they
`exercised with the first leg again for 30 min but now at 85% of Wmax
`(44 ⫾ 2 watts, heart rate 107 ⫾ 4 beats/min). Selection of the leg
`eligible for low-high respective moderate intensity was done by
`randomized stratification such that dominant and nondominant legs
`were similarly represented.
`Both during the resting and the exercise periods, subjects were
`sitting in a chair with the torso strapped to the back of the chair.
`Subjects had free access to water throughout the experiment. Pulmo-
`nary oxygen uptake and carbon dioxide excretion were measured
`regularly during exercise using an automated on-line system (Oxycon
`Champion; Erich Jaeger, Hoechberg, Germany).
`Microdialysis. Microdialysis was performed in principle as de-
`scribed previously (27). After anesthesia of the skin (0.2 ml lidocaine,
`5 mg/ml) at the sites of perforation, two microdialysis catheters (CMA
`60; CMA/Microdialysis, Stockholm, Sweden) were placed in the
`SCAT adjacent to the vastus lateralis part of the quadriceps muscle in
`each thigh. Catheters were placed equidistant from the skin and the
`muscle fascia, 13–15 cm above the patella, and with 2–3 cm between
`catheters. Catheters were perfused at a rate of 1.0 l/min using
`high-precision syringe pumps (CMA 100; CMA/Microdialysis). The
`perfusate consisted of Ringer acetate with 2 mM glucose, 5 kBq/ml
`[3H]glycerol (NEN, Zaventem, Belgium), and 5 kBq/ml [14C]ethanol
`(NEN). Seven out of 40 catheters ceased to function at some point
`during exercise.
`The in vivo relative recovery (RR) for glycerol was determined by
`the internal reference calibration technique (24). This technique as-
`sumes that the relative loss of [3H]glycerol from the microdialysis
`catheter to the interstitial fluid equals the RR of glycerol from the
`interstitial fluid to the microdialysis catheter. The RR of glycerol was
`calculated as (dpmp ⫺ dpmd)/dpmp, where dpmp is 3H disintegrations
`per minute in 5 l perfusate and dpmd is 3H disintegrations per minute
`
`in 5 l dialysate. Interstitial concentrations were calculated as (Cd ⫺
`Cp)/RR ⫹ Cp, where Cd is dialysate concentration and Cp is perfusate
`concentration.
`SCAT blood flow. Adipose tissue blood flow (ATBF) was measured
`by the local 133Xe washout technique (27), which has the advantage
`that it is a quantitative method. At least 30 min before start of the
`resting period, 0.5–1 MBq gaseous 133Xe (Amersham Health) in a
`volume of 0.05– 0.1 ml was injected in SCAT adjacent to the rectus
`femoris part of the quadriceps muscle. The 133Xe depot was placed
`equidistant from the skin and the muscle fascia and 13–15 cm above
`the patella. The washout rate of 133Xe was measured continuously by
`a scintillation counter system (Oakfield Instruments, Eynsham, UK)
`strapped to the skin surface above the 133Xe depot. ATBF was
`determined in 15- or 30-min periods and calculated as ⫺k 䡠 䡠 100
`(ml 䡠 100 g⫺1 䡠 min⫺1), where k is the rate constant of the washout and
` is the tissue-to-blood partition coefficient for 133Xe at equilibrium.
` was assumed to be 10 ml/g (15).
`Changes in ATBF in areas surrounding microdialysis catheters
`were also estimated by the microdialysis outflow/inflow technique
`(13, 25). The technique was originally described using ethanol as a
`marker of blood flow (13), but we have demonstrated that [14C]etha-
`nol is a valid alternative to ethanol (25). The blood flow marker is
`added to the perfusate (inflow), it diffuses out through the membrane,
`and is washed away by the blood. The higher the blood flow, the more
`marker is washed away, and the less marker remains in the dialysate
`(outflow). Accordingly, the outflow-to-inflow ratio of marker varies
`inversely with blood flow (9, 25). The advantage of the microdialysis
`outflow/inflow technique is that it estimates changes in blood flow in
`the same area as interstitial concentrations of metabolites are mea-
`sured.
`Sampling and analyses. Dialysate for analysis of glycerol was
`collected in 200-l capped microvials at 15- or 30-min intervals,
`immediately frozen, and kept at ⫺20°C until analysis. Dialysate
`sampling was delayed by 2 min relative to the rest of the experimental
`protocol to compensate for the transit time in the outlet tubing. Blood
`for determination of hormones and metabolites was sampled in iced
`tubes and immediately centrifuged. Blood was sampled immediately
`before and every 15 min during the first 30 min of the exercise periods
`and additionally at time 60 and 120 min during the 120-min exercise
`period. Blood for determination of glycerol was stabilized with
`EDTA, and plasma was kept at ⫺20°C until analysis. Microdialysate
`and plasma glycerol concentrations were determined by a CMA 600
`microdialysis analyzer (CMA/Microdialysis). Blood for determina-
`tion of insulin was stabilized with trasylol and EDTA and kept at
`⫺20°C until analysis. Arterial plasma insulin concentrations were
`determined by a commercial ELISA (DakoCytomation). Blood for
`determination of epinephrine was stabilized with reduced glutathione
`and EDTA and kept at ⫺80°C until analysis. Arterial plasma epineph-
`rine concentrations were determined by a commercial RIA (High
`sensitive 2 CAT RIA; Labor Diagnostika, Nord, Germany).
`Calculation of adipose tissue lipolysis. Adipose tissue venous
`glycerol concentrations were calculated based on “Ficks law of
`
`Fig. 1. Experimental protocol. Ten males performed knee exten-
`sion exercise with one leg (leg 1) at 25% of maximal workload
`(Wmax) for 30 min while the other leg (leg 2) was resting. After a
`short rest, subjects exercised with the other leg (leg 2) at 55%
`Wmax for 120 min while the first leg (leg 1) was resting. After
`another short rest, subjects exercised with the first leg (leg 1) at
`85% Wmax for 30 min while the other leg (leg 2) was resting.
`Arterial blood samples were obtained as indicated with arrows,
`whereas microdialysis samples and 133Xe washout readings were
`collected across sampling periods indicated by brackets.
`
`AJP-Endocrinol Metab • VOL 292 • FEBRUARY 2007 • www.ajpendo.org
`Downloaded from journals.physiology.org/journal/ajpendo (038.099.035.066) on November 24, 2021.
`
`LUMENIS EX1084
`Page 2
`
`
`
`E396
`
`SPOT LIPOLYSIS
`
`RESULTS
`
`ATBF. Knee extension exercise with one leg at 25, 55, and
`85% Wmax increased (P ⬍ 0.05) femoral subcutaneous ATBF
`in both legs (Fig. 2A). In general, blood flow was higher in
`adipose tissue adjacent to working muscle than in adipose
`tissue adjacent to resting muscle. At 25 and 55% Wmax, the
`ATBF difference between legs (rest vs. exercise) was signifi-
`cant, but this was not the case at 85% Wmax. The changes in
`ATBF detected by the microdialysis [14C]ethanol technique
`were similar to changes detected by the 133Xe washout tech-
`nique (Fig. 2, A and B).
`Adipose tissue interstitial and arterial plasma glycerol con-
`centrations. Knee extension exercise with one leg at 55 and
`85% Wmax increased (P ⬍ 0.05) the femoral SCAT interstitial
`glycerol concentration compared with rest (Fig. 3A). At 85%
`Wmax, the interstitial glycerol concentration was higher (P ⬍
`0.05) in adipose tissue adjacent to working muscle than in
`adipose tissue adjacent to resting muscle. At all three intensi-
`ties, knee extension exercise with one leg increased (P ⬍ 0.05)
`the arterial plasma glycerol concentration compared with rest
`(Fig. 3B).
`Adipose tissue lipolysis. During knee extension exercise
`with one leg at 25 and 85% Wmax, the lipolysis was signifi-
`cantly higher in femoral SCAT adjacent to working muscle
`than in adipose tissue adjacent to resting muscle (Fig. 4).
`During the first 60 min of exercise with one leg at 55% Wmax,
`the lipolysis seemed higher in adipose tissue adjacent to work-
`ing muscle than in adipose tissue adjacent to resting muscle,
`but the difference was not significant. The difference in adi-
`pose tissue lipolysis between the two legs (lipolysis in exer-
`cising ⫺ resting leg) during time 0 –30 min showed that
`lipolysis was larger (P ⬍ 0.05) than zero at 25 and 85% Wmax,
`but not at 55% Wmax (Fig. 5). Incremental lipolysis did not
`change with exercise intensity (P ⬎ 0.05).
`Arterial plasma hormone concentrations. Knee extension
`exercise with one leg at 55% and at 85% Wmax decreased (P ⬍
`0.05) the arterial plasma insulin concentration compared with
`rest (Table 1). Exercise with one leg at 85% Wmax increased
`(P ⬍ 0.05) the arterial plasma epinephrine concentration com-
`pared with rest (Table 1).
`
`DISCUSSION
`
`Specific exercises can induce spot lipolysis, since we found
`blood flow (Fig. 2) and lipolysis (Figs. 4 and 5) to be higher in
`SCAT adjacent to contracting than adjacent to resting muscle.
`Based on the present results, it cannot be foreseen if specific
`exercises can induce spot reduction, since triacylglycerol (TG)
`stores could be fully replenished or even supercompensated
`between exercise sessions.
`During exercise, temperature increases in the contracting
`muscles (10), and this would increase temperature also in the
`tissues adjacent to the contracting muscles. An increase in
`adipose tissue temperature induces an increase in ATBF (9),
`and this mechanism could potentially explain the increased
`blood flow in the adipose tissue adjacent to the contracting
`muscle in the present study (Fig. 2). During knee extension
`exercise, the increase in temperature of the contracting quad-
`riceps muscle has been found to be ⬃2°C (10). Fella¨nder et al.
`(9) heated SCAT and increased the temperature in the tissue by
`4°C, which elicited an increase in ATBF of ⬃1.5 ml 䡠100
`
`Fig. 2. Adipose tissue blood flow. Ten healthy, overnight-fasted males per-
`formed one-legged knee extension exercise at 25% of Wmax for 30 min
`followed by exercise at 55% Wmax for 120 min with the other leg and finally
`exercised at 85% Wmax for 30 min with the first leg. Subjects rested for 30 min
`between exercise periods. Femoral, subcutaneous adipose tissue blood flow of
`both legs was measured using the 133Xe washout (A) and the microdialysis
`[14C]ethanol outflow/inflow (B) techniques. Adipose tissue blood flow is
`inversely related to [14C]ethanol outflow/inflow. *P ⬍ 0.05 vs. rest. (*)P ⬍ 0.1
`vs. rest. ⫹P ⬍ 0.05 between legs. (⫹)P ⬍ 0.1 between legs.
`
`diffusion for a thin membrane,” as described previously (27). Subse-
`quently, adipose tissue glycerol output was calculated as venous
`minus arterial glycerol concentration multiplied by ATBF (27). Adi-
`pose tissue glycerol output equals adipose tissue lipolysis, since
`glycerol is not reused to any significant extent in adipose tissue (3).
`Statistics. The computer program SigmaStat for Windows version
`2.03 (SPPS, Chicago, IL) was used for statistical analysis. All data are
`presented as means ⫾ SE. A two-way repeated-measures ANOVA
`(RM ANOVA) with leg and time as factors was used to test for
`differences between legs and changes with time in ATBF and micro-
`dialysis-associated variables. A one-way RM ANOVA was used to
`test for changes with time in plasma concentrations. If a difference
`was present according to the ANOVA, a Student-Newman-Keul’s test
`was used post hoc to locate the difference. A one-sample t-test was
`used to test if incremental lipolysis differed from zero. A significance
`level of 0.05 in two-tailed testing was chosen a priori.
`
`AJP-Endocrinol Metab • VOL 292 • FEBRUARY 2007 • www.ajpendo.org
`Downloaded from journals.physiology.org/journal/ajpendo (038.099.035.066) on November 24, 2021.
`
`LUMENIS EX1084
`Page 3
`
`
`
`SPOT LIPOLYSIS
`
`E397
`
`Fig. 4. Adipose tissue lipolysis. Ten healthy, overnight-fasted males per-
`formed one-legged knee extension exercise at 25% of Wmax for 30 min
`followed by exercise at 55% Wmax for 120 min with the other leg and finally
`exercised at 85% Wmax for 30 min with the first leg. Subjects rested for 30 min
`between exercise periods. Femoral, subcutaneous adipose tissue lipolysis of
`both legs was calculated from the interstitial and arterial glycerol concentra-
`tions and adipose tissue blood flow. *P ⬍ 0.05 vs. rest. ⫹P ⬍ 0.05 between
`legs. (⫹)P ⬍ 0.1 between legs.
`
`This would increase the interstitial concentration of epineph-
`rine in the adipose tissue, which could be one of the mecha-
`nisms behind the higher lipolysis in SCAT adjacent to con-
`tracting than adjacent to resting muscle.
`Norepinephrine is not only a hormone but also a neurotrans-
`mitter in the sympathetic nervous system, and a selective
`stimulation of adipose tissue adjacent to contracting muscles
`could also be via local sympathetic nerves. In favor of this
`mechanism, studies in humans have demonstrated that regional
`differences are present in sympathetic nervous activity (5) and
`that direct stimulation of a cutaneous sympathetic nerve in-
`
`Fig. 3. Glycerol concentrations. Ten healthy, overnight-fasted males per-
`formed one-legged knee extension exercise at 25% of Wmax for 30 min
`followed by exercise at 55% Wmax for 120 min with the other leg and finally
`exercised at 85% Wmax for 30 min with the first leg. Subjects rested for 30 min
`between exercise periods. The interstitial glycerol concentration in femoral,
`subcutaneous adipose tissue of both legs was measured by microdialysis
`technique (A), and the plasma glycerol concentration was measured in arterial
`blood (B). P ⬍ 0.05 vs. rest (*) and between legs (⫹).
`
`g⫺1 䡠min⫺1. We did not measure the temperature of the adipose
`tissue adjacent to the contracting muscles, but, assuming that
`the adipose tissue temperature increased 1°C, this could ac-
`cording to the data by Fella¨nder et al. (9) at most explain an
`increase in ATBF of 0.4 ml 䡠100 g⫺1 䡠min⫺1.
`Circulating epinephrine and norepinephrine are potent stim-
`ulators of ATBF and lipolysis (17, 26), and, in the present
`study, we found the plasma epinephrine concentration to in-
`crease significantly at the highest exercise intensity (Table 1).
`Circulating hormones influence all adipose tissue depots and
`not selectively adipose tissue adjacent to contracting muscles.
`However, because of the relatively increased blood flow in
`adipose tissue adjacent to contracting muscles, a larger amount
`of the circulating epinephrine will be delivered to this tissue.
`
`Fig. 5. Incremental adipose tissue lipolysis. Ten healthy, overnight-fasted
`males performed one-legged knee extension exercise at 25% of Wmax for 30
`min followed by exercise at 55% Wmax for 120 min with the other leg and
`finally exercised at 85% Wmax for 30 min with the first leg. Subjects rested for
`30 min between exercise periods. The difference in mean femoral, subcutane-
`ous adipose tissue lipolysis between exercising and resting legs was calculated
`for the time period 0 –30 min for each exercise period (25, 55, and 85% Wmax).
`*P ⬍ 0.05 vs. 0.
`
`AJP-Endocrinol Metab • VOL 292 • FEBRUARY 2007 • www.ajpendo.org
`Downloaded from journals.physiology.org/journal/ajpendo (038.099.035.066) on November 24, 2021.
`
`LUMENIS EX1084
`Page 4
`
`
`
`E398
`
`SPOT LIPOLYSIS
`
`Table 1. Arterial plasma hormone concentrations
`
`Exercise
`
`Time, min
`
`Insulin, pM
`
`Epinephrine, nM
`
`Rest
`25% Wmax
`
`55% Wmax
`
`85% Wmax
`
`Rest
`15
`30
`15
`30
`60
`120
`15
`30
`
`45⫾6
`41⫾8
`37⫾5
`35⫾5*
`31⫾4*
`30⫾4*
`25⫾3*
`29⫾5*
`31⫾4*
`
`1.1⫾0.3
`0.8⫾0.1
`0.9⫾0.2
`0.8⫾0.1
`0.8⫾0.1
`0.7⫾0.1
`0.8⫾0.1
`1.1⫾0.3
`1.3⫾0.1*
`
`Values are means ⫾ SE. Wmax, maximal workload. *P ⬍ 0.05 vs. rest.
`
`creases lipolysis in the adipose tissue innervated by the stim-
`ulated nerve (6). Also, denervation of a rat retroperitoneal fat
`pad induces an increase in fat pad weight and adipocyte
`volume 1 wk after denervation, indicating that lipolysis is
`diminished when no sympathetic innervation is present (4).
`Another mechanism to explain the increase in ATBF (Fig. 2)
`and lipolysis (Figs. 4 and 5) adjacent to contracting muscles
`could be release of paracrine substances from the contracting
`muscles, which could diffuse from the muscle to the adipose
`tissue to stimulate blood flow and lipolysis. Contracting skel-
`etal muscle has been shown to release the myokine interleu-
`kin-6 (8), which among other things has been shown to stim-
`ulate adipose tissue lipolysis (18).
`It is evident that several potential mechanisms can explain
`the increased ATBF (Fig. 2) and lipolysis (Figs. 4 and 5) in
`adipose tissue adjacent to contracting muscles, but it is more
`difficult
`to understand the rationale for the increase. The
`muscle and the SCAT superficial to the muscle have separate
`blood supplies and drainages. Accordingly, fatty acids released
`from adipose tissue during exercise are transported to the heart
`and reach all parts of the body, not specifically the muscle
`beneath the subcutaneous tissue from which the fatty acids
`were released.
`In the present study, we measured changes in ATBF by two
`independent methods, the 133Xe washout technique (27) and
`the microdialysis [14C]ethanol outflow/inflow technique (25),
`and findings were similar (Fig. 2). Both methods showed a
`general increase in ATBF during exercise and that blood flow
`was higher in adipose tissue adjacent to contracting muscle
`than in adipose tissue adjacent to resting muscle. The 133Xe
`washout and the microdialysis ethanol outflow/inflow tech-
`niques have previously shown comparable results in adipose
`tissue during external heating of the skin (9).
`We found lipolysis to be higher in adipose tissue adjacent to
`contracting muscles than in adipose tissue adjacent to resting
`muscle (Figs. 4 and 5), but, in contrast to findings for ATBF
`(Fig. 2), we did not find a general exercise-induced increase in
`femoral adipose tissue lipolysis. Cycle exercise of moderate
`intensity increases lipolysis in abdominal SCAT (2, 14), but, in
`accordance with our findings, Horowitz et al. (14) found that
`exercise at 50% of V˙ O2 max did not increase femoral SCAT
`lipolysis. We examined femoral SCAT because this tissue has
`a contralateral tissue site that can be used as a control, which
`abdominal SCAT clearly do not.
`Reliability of techniques used in the study should be con-
`sidered. We placed the microdialysis probes and the 133Xe
`depot in the femoral SCAT equidistant from skin and muscle
`
`fascia. We did not measure the thickness of the femoral SCAT,
`but,
`in studies of similar subjects, we found the femoral
`skinfold to be ⬃10 –30 mm (unpublished results). We believe
`that measurements of lipolysis and blood flow in adipose tissue
`depots of this thickness are reliable. Theoretical predictions (7)
`suggest that a microdialysis probe estimates the metabolite
`concentration in a radius of a few millimeters from the probe,
`and probes thus should mirror adipose tissue metabolism and
`not metabolism in skin or muscle. In theory, also the 133Xe
`depot could come in contact with skin or muscle, but, if this
`had occurred, the washout curve for 133Xe would have become
`multiexponential, and this was not the case.
`More calories are expended during aerobic, whole body
`exercise than by exercise with local muscle groups, and,
`accordingly, a person seeking to loose fat must be advised to
`perform whole body exercise. However, the present study has
`shown that blood flow (Fig. 2) and lipolysis (Figs. 4 and 5) are
`stimulated more in adipose tissue adjacent to contracting mus-
`cles than in adipose tissue adjacent to resting muscles. The
`incremental femoral SCAT lipolysis (Fig. 5) showed no clear
`connection with exercise intensity and amounted to 22– 80
`nmol 䡠100 g⫺1 䡠min⫺1 during the first 30 min of the exercise
`bout. Assuming a molecular weight of 860 g/mol for TG, this
`corresponds to an extra breakdown of 0.6 –2.1 mg of TG in 30
`min/100 g of adipose tissue adjacent to contracting muscles.
`These figures are comparable to the increase in lipolysis
`induced by cycle exercise at 50% of V˙ O2 max, which was found
`to be ⬃50 nmol 䡠100 g⫺1 䡠min⫺1 in femoral SCAT and 200
`nmol 䡠100 g⫺1 䡠min⫺1 in abdominal SCAT (time 15– 40 min;
`see Ref. 14), corresponding to a breakdown of 1.3 and 5.2 mg
`of TG in 30 min/100 g of femoral and abdominal adipose
`tissue, respectively.
`In conclusion, an acute bout of exercise can induce spot
`lipolysis and increased blood flow in adipose tissue adjacent to
`contracting skeletal muscle.
`
`ACKNOWLEDGMENTS
`
`We highly appreciate the expert technical assistance of Thomas Beck, Jeppe
`Bach, and Regitze Kraunsøe.
`
`GRANTS
`
`The study was supported by The Danish Ministry of Culture Committee on
`Sports Research.
`
`REFERENCES
`
`1. Andersen P, Adams RP, Sjøgaard G, Thorboe A, Saltin B. Dynamic
`knee extension as model for study of isolated exercising muscle in
`humans. J Appl Physiol 59: 1647–1653, 1985.
`2. Arner P, Kriegholm E, Engfeldt P, Bolinder J. Adrenergic regulation of
`lipolysis in situ at rest and during exercise. J Clin Invest 85: 893– 898,
`1990.
`3. Arner P, Liljeqvist L, O¨ stman J. Metabolism of mono- and diacylglyc-
`erols in subcutaneous adipose tissue of obese and normal-weight subjects.
`Acta Med Scand 200: 187–194, 1976.
`4. Cousin B, Casteilla L, Lafontan M, Ambid L, Langin D, Berthault
`MF, Penicaud L. Local sympathetic denervation of white adipose tissue
`in rats induces preadipocyte proliferation without noticeable changes in
`metabolism. Endocrinology 133: 2255–2262, 1993.
`5. Cox HS, Kaye DM, Thompson JM, Turner AG, Jennings GL, Itsio-
`poulos C, Esler MD. Regional sympathetic nervous activation after a
`large meal in humans. Clin Sci Colch 89: 145–154, 1995.
`6. Dodt C, Lo¨nnroth P, Fehm HL, Elam M. Intraneural stimulation elicits
`an increase in subcutaneous interstitial glycerol
`levels in humans.
`J Physiol Lond 521: 545–552, 1999.
`
`AJP-Endocrinol Metab • VOL 292 • FEBRUARY 2007 • www.ajpendo.org
`Downloaded from journals.physiology.org/journal/ajpendo (038.099.035.066) on November 24, 2021.
`
`LUMENIS EX1084
`Page 5
`
`
`
`SPOT LIPOLYSIS
`
`E399
`
`7. Dykstra KH, Hsiao JK, Morrison PF, Bungay PM, Mefford IN, Scully
`MM, Dedrick RL. Quantitative examination of tissue concentration
`profiles associated with microdialysis. J Neurochem 58: 931–940, 1992.
`8. Febbraio MA, Pedersen BK. Contraction-induced myokine production
`and release: is skeletal muscle an endocrine organ? Exerc Sport Sci Rev
`33: 114 –119, 2005.
`9. Fella¨nder G, Linde B, Bolinder J. Evaluation of the microdialysis
`ethanol technique for monitoring of subcutaneous adipose tissue blood
`flow in humans. Int J Obes 20: 220 –226, 1996.
`10. Ferguson RA, Ball D, Krustrup P, Aagaard P, Kjaer M, Sargeant AJ,
`Hellsten Y, Bangsbo J. Muscle oxygen uptake and energy turnover
`during dynamic exercise at different contraction frequencies in humans.
`J Physiol 536: 261–271, 2001.
`11. Garrow JS, Summerbell CD. Meta-analysis: effect of exercise, with or
`without dieting, on the body composition of overweight subjects. Eur
`J Clin Nutr 49: 1–10, 1995.
`12. Gwinup G, Chelvam R, Steinberg T. Thickness of subcutaneous fat and
`activity of underlying muscles. Ann Intern Med 74: 408 – 411, 1971.
`13. Hickner RC, Rosdahl H, Borg I, Ungerstedt U, Jorfeldt L, Henriksson
`J. Ethanol may be used with the microdialysis technique to monitor blood
`flow changes in skeletal muscle: dialysate glucose concentration is blood-
`flow-dependent. Acta Physiol Scand 143: 355–356, 1991.
`14. Horowitz JF, Leone TC, Feng W, Kelly DP, Klein S. Effect of
`endurance training on lipid metabolism in women: a potential role for
`PPARalpha in the metabolic response to training. Am J Physiol Endocrinol
`Metab 279: E348 –E355, 2000.
`15. Jansson PA, Lo¨nnroth P. Comparison of two methods to assess the
`tissue/blood partition coefficient for xenon in subcutaneous adipose tissue
`in man. Clin Physiol 15: 47–55, 1995.
`16. Krotkiewski M, Aniansson A, Grimby G, Bjo¨rntorp P, Sjo¨stro¨m L.
`The effect of unilateral isokinetic strength training on local adipose and
`muscle tissue morphology, thickness, and enzymes. Eur J Appl Physiol 42:
`271–281, 1979.
`17. Kurpad A, Khan K, Macdonald I, Elia M. Haemodynamic responses
`in muscle and adipose tissue and whole body metabolic responses
`
`during norepinephrine infusions in man. J Auton Nerv Syst 54: 163–
`170, 1995.
`18. Lyngsø D, Simonsen L, Bu¨ low J. Metabolic effects of interleukin-6 in
`human splanchnic and adipose tissue. J Physiol 543: 379 –386, 2002.
`19. Olson AL, Edelstein E. Spot reduction of subcutaneous adipose tissue.
`Res Q 39: 647– 652, 1968.
`20. Roby FB. Effect of exercise on regional subcutaneous fat accumulations.
`Res Quar 33: 273–278, 1962.
`21. Ross R, Dagnone D, Jones PJ, Smith H, Paddags A, Hudson R,
`Janssen I. Reduction in obesity and related comorbid conditions after
`diet-induced weight
`loss or exercise-induced weight
`loss in men. A
`randomized, controlled trial. Ann Intern Med 133: 92–103, 2000.
`22. Ross R, Janssen I. Physical activity, total and regional obesity: dose-
`response considerations. Med Sci Sports Exerc 33: S521–S527, 2001.
`23. Ross R, Janssen I, Stallknecht B. Influence of endurance exercise on
`adipose tissue distribution. In: Endurance Exercise and Adipose Tissue,
`edited by Nicklas BJ. Boca Rat
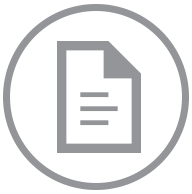
Accessing this document will incur an additional charge of $.
After purchase, you can access this document again without charge.
Accept $ ChargeStill Working On It
This document is taking longer than usual to download. This can happen if we need to contact the court directly to obtain the document and their servers are running slowly.
Give it another minute or two to complete, and then try the refresh button.
A few More Minutes ... Still Working
It can take up to 5 minutes for us to download a document if the court servers are running slowly.
Thank you for your continued patience.
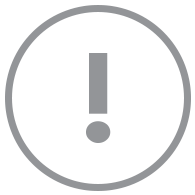
This document could not be displayed.
We could not find this document within its docket. Please go back to the docket page and check the link. If that does not work, go back to the docket and refresh it to pull the newest information.
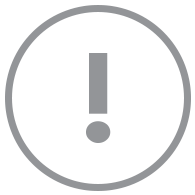
Your account does not support viewing this document.
You need a Paid Account to view this document. Click here to change your account type.
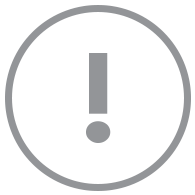
Your account does not support viewing this document.
Set your membership
status to view this document.
With a Docket Alarm membership, you'll
get a whole lot more, including:
- Up-to-date information for this case.
- Email alerts whenever there is an update.
- Full text search for other cases.
- Get email alerts whenever a new case matches your search.
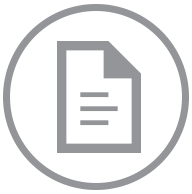
One Moment Please
The filing “” is large (MB) and is being downloaded.
Please refresh this page in a few minutes to see if the filing has been downloaded. The filing will also be emailed to you when the download completes.
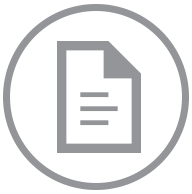
Your document is on its way!
If you do not receive the document in five minutes, contact support at support@docketalarm.com.
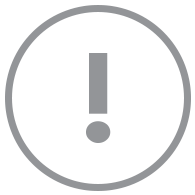
Sealed Document
We are unable to display this document, it may be under a court ordered seal.
If you have proper credentials to access the file, you may proceed directly to the court's system using your government issued username and password.
Access Government Site