`
`Anal. Cham. 1992, 64, 967-972
`temperatures above 200 °C. The cause of the discoloration
`is not known but resulted in the need to heat the vapor cell
`to successively higher temperatures each time it was used in
`order to totally absorb the laser scatter.
`Raman Spectra with the Hg Vapor Cell. The pulsed
`Raman spectra of ethanol with excitation at 253.65 nm is
`shown in Figures 3a (no Hg cell) and 3b (Hg cell, 250 °C, in
`place). Just as in the case of the Rb filter, the laser scatter
`was completely eliminated by absorption in the metal vapor.
`However, in this case, the resolution of the monochromator
`system (1-nm spectral bandpass, about 150 cm"1) was insuf-
`ficient to resolve the two closely spaced peaks at 1100 cm'1.
`In Figures 4a and 4b are shown the Raman spectra of a solid
`crystal of potassium nitrate with and without the use of the
`In Figure 4a the laser scatter is so dominant
`Hg vapor filter.
`that the Raman peaks at 1055 and 1350 cm'1 are obscured.
`In Figure 4b, these two peaks are clearly evident after at-
`tenuation of stray light by the Hg filter. The small peak at
`3600 cm'1 in Figure 4a is an artifact and does not appear in
`Figure 4b.
`
`ACKNOWLEDGMENT
`This research was supported by a grant from the National
`Institutes of Health: NIH-5-R01-GM 38434-04.
`REFERENCES
`(1) Pelletier, M. J. Appl. Spectrosc. 1990, 44, 1699.
`(2) Woodward, L. A. In Raman Spectroscopy : Theory and Practice : Vol.
`2, H. A. Szymanski, ed.; Plenum Press: New York, 1970; Vol. 2.
`Inc.: New York
`(3) Long, D. A. Raman Spectroscopy, McGraw-Hill,
`1977.
`(4) Devlin, G. E.; Davis, J. L.; Chase, L.; Geschwind, S. Appl. Phys. Lett.
`1971, 19, 138.
`(5) Schoen, P. E.; Jackson, D. A. J. Phys. E. 1972, 5, 519.
`(6) Wall, K. F.; Chang, R. K. Opt. Lett. 1986, 11, 493.
`(7) Grossman, J. J. Filter Spectrograph. U.S. Patent No. 3,865,490,
`1975.
`(8) Huang, Y.; Yu, P. Y. Rev. Scl. Instrum. 1988, 59, 190.
`(9) Gowers, C.; Hirsch, K.; Nielson, P.; Salzman, H. Appl. Opt. 1988, 27,
`3625.
`(10) Carlson, R. J.; Asher, S. A. Appl. Spectrosc. 1984, 38, 297.
`(11) Asher, S. A.; Flaugh, P. L.; Washinger, G. Spectroscopy 1986, 1, 26.
`(12) Flaugh, P. L.; O’Donnell, S. E.; Asher, S. A. Appl. Spectrosc. 1984,
`38, 847.
`(13) Carrabba, M. M.; Spencer, K. M.; Rich, C.; Rauh, D. Appl. Spectrosc.
`1990, 44, 1558.
`(14) Yang, B.; Morris, M. D.; Owen, H. Appl. Spectrosc. 1991, 45, 1533.
`(15) Chou, P. T.; Studer, S. L.; Martinez, M. L. Appl. Spectrosc. 1991, 45,
`513.
`(16) Hamaguchi, H.; Kamogawa, K. Appl. Spectrosc. 1986, 40, 564.
`(17) Puppels, G. J.; Huizinga, A.; Krabbe, H. W.; de Boer, H. A.; Gijsbers,
`G.; de Mul, F. F. M. Rev. Scl. Instrum. 1990, 61, 3709.
`(18) Smrthells, C. J. Metals Reference Book, Vol. II: Butterworths: Lon-
`don, 1962; p 655.
`(19) Radzig, A. A.; Smirnov, B. M. Reference Data on Atoms, Molecules
`and Ions: Springer-Verlag: Berlin, 1985.
`(20) Alkemade, C. Th. J.; Hollander, Tj.; Snellman, W.; Zeegers, P. J. Th.
`Metal Vapours In Flames: Pergamon Press: Oxford, 1982.
`
`Received for review November 4,1991. Accepted January
`27, 1992.
`
`CONCLUSIONS
`The Rb and Hg metal vapor filters were shown to be ef-
`It is clear
`fective in eliminating laser scatter Raman spectra.
`that further work must be done to study the stability of these
`filters with time at elevated temperatures and to examine their
`practical use with more optimized Raman spectrometers.
`When used in conjunction with a single mode diode laser,
`locked, for example, onto the cesium transition at 852.10 nm,
`and a compact, high-efficiency spectrometer with diode array
`or CCD detection, such filters may make possible more
`sen-
`sitive and certainly more compact Raman instrumentation.
`
`Capillary Array Electrophoresis Using Laser-Excited Confocal Fluorescence Detection
`
`Xiaohua C. Huang, Mark A. Quesada, and Richard A. Mathies*
`Department of Chemistry, University of California, Berkeley, California 94720
`INTRODUCTION
`large surface-to-volume ratio of the capillary channel, the use
`of thin capillary walls (50-150 Mm), and the high thermal
`Capillary electrophoresis (CE) has found widespread ap-
`conductivity of the wall material.1
`plication in analytical and biomedical research, and the scope
`Although CE provides rapid analysis, thus far the total
`and sophistication of CE is still rapidly advancing.1"5 Gel-filled
`throughput is not high because only one capillary can be
`capillaries have been employed for the rapid separation and
`analyzed at a time. Developing a method to increase the
`analysis of synthetic polynucleotides,6 DNA sequencing
`throughput of CE is a challenging and important task. One
`fragments,7"11 and DNA restriction fragments.12,13 Open-tube
`possible approach is to employ higher electric fields which
`capillary electrophoresis has attained subattomole detection
`would provide faster separations. Higher electric fields,
`levels in amino acid separations14 and proven its utility for
`however, often introduce overheating of the columns and
`the separation of proteins, viruses, and bacteria.15 Separation
`column failure. Another way to increase the throughput is
`of the optical isomers of dansyl amino acids has also been
`to run a large number of capillary separations in parallel. This
`successfully demonstrated.16 Micellar electrokinetic capillary
`approach uses an array of capillaries and is therefore called
`chromatography, isoelectric focusing, and on-column deriva-
`capillary array electrophoresis (CAE). CAE is potentially
`tization can all be performed on CE columns, demonstrating
`advantageous because the individual capillaries can be inde-
`the utility of capillary electrophoresis as an analytical and
`pendently manipulated at the inlet, thereby facilitating rapid,
`micropreparative tool.4,5
`In our approach, the
`parallel loading of multiple samples.
`The advantages of CE arise intrinsically from the use of
`capillaries are combined into a ribbon at the outlet for ease
`a small inside diameter (20-200 Mm) capillary. High electric
`In this way, a 2 order of
`of parallel, on-column detection.
`fields can be applied along small diameter fused-silica cap-
`magnitude increase in CE throughput should be achieved
`illaries without a significant increase in temperature. Since
`because hundreds of capillaries can be easily bundled for
`the electrophoretic velocity of the charged species is pro-
`detection.
`portional to the applied field, CE can achieve rapid, high-
`An important problem confronting capillary array elec-
`resolution separation. The reduced Joule heating in CE is
`trophoresis is detection. Since small amounts of sample are
`a result of the low current passing through the capillary, the
`injected in a capillary, a high-sensitivity detection system is
`indispensable. Laser-excited fluorescence has proven to be
`a sensitive detection method in capillary electrophoresis and
`
`* To whom correspondence and reprint requests should be ad-
`dressed.
`
`0003-2700/92/0364-0967$03.00/0
`
`© 1992 American Chemical Society
`
`Downloaded via UNIV OF VIRGINIA on November 6, 2018 at 18:18:25 (UTC).
`
`See https://pubs.acs.org/sharingguidelines for options on how to legitimately share published articles.
`
`Agilent Exhibit 1266
`Page 1 of 6
`
`
`
`968 • ANALYTICAL CHEMISTRY, VOL. 64, NO. 8, APRIL 15, 1992
`
`High Voltage Power Supply
`
`Laser light (1 mW, 488 nm at sample) is reflected by
`Figure 1. Schematic of the laser-excited, confocal fluorescence capillary array scanner.
`a long-pass dichroic beam splitter, passed through a 32X, N.A. 0.4 microscope objective, and brought to a focus within a 100-^m-i.d. capillary.
`The resulting fluorescence is collected by the objective, passed back through the beam splitter, and focused on a 400-um-diameter confocal
`pinhole. After the spatial filter, the emission is spectrally filtered and detected with a photomultiplier. The output is amplified, digitized, and then
`stored in a computer. A computer-controlled stage with 2.5-gm resolution is used to translate the capillary array past the optical system.
`
`in DNA sequencing.7'11,14,17'21 In most
`laser-excited fluores-
`cence detection schemes, the incident laser beam and the
`It is
`emitted fluorescence are perpendicular to each other.
`difficult to configure a system to detect an array of capillaries
`using this 90° geometry. We have recently introduced a la-
`ser-excited, confocal fluorescence gel scanner which provides
`enhanced detection of fluorescently labeled DNA in slab
`gels.22'26 This detection system utilizes an epi-illumination
`format where the laser is focused on the sample by a micro-
`scope objective and the emitted fluorescence is gathered by
`the same objective using a 180° geometry followed by confocal
`detection. This geometry is ideal for on-column detection of
`capillaries. Using confocal excitation and detection, the depth
`of field of the optical system is sufficiently small that only
`the interior of the capillary is probed. Background scattering
`and reflections from the capillary wall are rejected by the
`spatial filter and by spectroscopic filters. The utility of
`fluorescence microscope detection for CE has been recognized
`in previous studies utilizing single capillaries.21,28 We show
`can be used to detect an array of
`here that confocal scanners
`capillary columns with high sensitivity.
`EXPERIMENTAL SECTION
`Scanning Confocal Fluorescence Detection. Confocal
`fluorescence detection of capillary arrays is performed with the
`system shown in Figure 1. An argon ion laser (Model 2020,
`Spectra-Physics, Mountain View, CA) is used for excitation. The
`laser beam is expanded to 5-mm diameter, collimated, and then
`reflected through a 32X, N.A. 0.4 infinite conjugate objective (LD
`Plan-Achromat 440850, Carl Zeiss, West Germany) by a long-pass
`dichroic beam splitter (480DM, Omega Optical, Brattleboro, VT).
`This dichroic beam splitter reflects the excitation laser beam but
`transmits fluorescent light which is Stoke’s shifted to longer
`wavelengths. The objective focuses the exciting laser on the
`
`sample and gathers the fluorescence with very high collection
`efficiency. The use of an infinite conjugate objective permits
`vertical adjustment of the probe volume by translating the ob-
`jective with no significant perturbation of the optical alignment.
`The focused 1-mW beam at 488 nm had a 9-^m beam diameter
`and a 25->im confocal beam parameter. The fluorescence emission
`is passed back through the long-pass dichroic beam splitter to
`reduce laser interference and to separate the excitation and de-
`tection paths. The fluorescence is then focused by a 75-mm focal
`length lens on a 400-Mm pinhole which serves
`as the confocal
`spatial filter. The light passing through the pinhole is filtered
`by a 488-nm rejection band filter (488 RB filter, Omega Optical,
`Brattleboro, VT), a long-pass cutoff filter (Schott GG-495, Esco,
`Oakridge, NJ), and a fluorescence bandpass filter (530 DF60,
`Omega Optical, Brattleboro, VT) followed by detection with a
`cooled photomultiplier tube (RCA 31034A, Burle Industries,
`Lancaster, PA). The output of the phototube was amplified and
`filtered with a low-noise amplifier (SR560, Stanford Research
`Systems, Sunnyvale, CA), digitized with a 12-bit analog-to-digital
`board (DASH-16F, Metra-Byte, Taunton, MA), and stored in an
`IBM PS/2 computer.
`Data Acquisition and Imaging. During electrophoresis, the
`translation stage (Model 4000, Design Components, Franklin, MA)
`is programmed to continuously scan the capillary array back and
`forth at 20 mm/s in a direction perpendicular to the electro-
`phoresis direction. The image acquired in this way has two
`dimensions. One is a spatial dimension representing the physical
`image of the capillaries. The other is a temporal dimension
`proportional to the elapsed time. During a particular sweep,
`fluorescence data are sampled at 2000 Hz so the nominal image
`resolution is 10 /xm/pixel; thus, 10 pixels represent the interior
`100-Min width of any given capillary. The electronic low-pass filter
`was set at 300 Hz to provide high-frequency noise rejection while
`still passing the spatial frequencies required to define the 100-fun
`i.d. of the capillaries. An image of the migrating bands is built
`up as a function of time by accumulating periodic 1-s sweeps of
`
`Agilent Exhibit 1266
`Page 2 of 6
`
`
`
`ANALYTICAL CHEMISTRY, VOL. 64, NO. 8, APRIL 15, 1992 • 969
`
`G G G G
`
`Figure 2. Exploded view of the capillary array holder. The holder was
`constructed from a ’/4-in.-thick 5- X 5-cm aluminum base plate and
`a V1(-in.-thlck 2- X 4-cm cover plate. The plates were held together
`by four screws, and there was a matching 4- X 20-mm slot milled in
`each. The capillaries were sandwiched between the base and cover
`such that the exposed windows could be viewed through the slot.
`
`the exposed region of the capillaries. The transit time of the
`migrating DNA past the probe region, under the conditions em-
`ployed here, ranges from approximately 10 s for the low molecular
`weight fragments (40-50 mers) to 14 s for the higher molecular
`weight fragments (380-390 mers). With l-s repeat cycles, this
`gives 10-14 data points for each band. The computer controls
`the translation stage and displays the acquired image in real time.
`Image processing was performed with the NIH program, Image
`1.29, and a commercial image processing package, Canvas. The
`image data were reduced to a one-dimensional line plot or elec-
`the width of each
`tropherogram by averaging the pixels across
`lane.
`Preparation of Capillary Gel Columns. Zero-cross-linked
`poly(acrylamide) gel-filled capillaries were prepared using a
`modified version of the procedure described by Cohen et al.6-7 A
`3-mm-wide detection window was produced in each lOO-jim-i.
`d./200-/xm-o.d. fused-silica capillary (Polymicro Technologies,
`Phoenix, AZ) by burning off the polyimide coating with a hot wire.
`The window was burned ~25 cm from the inlet side of the 40-
`cm-long capillary. The inner wall of the capillaries was
`then
`treated overnight with a bifunctional reagent, [y-(methacryl-
`oxy)propyl]trimethoxysilane to prepare the walls for acrylamide
`adhesion.6 Freshly-made acrylamide gel solution (9% T, 0% C)
`in a 1 x TBE buffer (Tris-boric acid-EDTA) with 7 M urea was
`filtered with an 0.2-/im syringe filter and degassed under vacuum
`for about 1 h. 10% TEMED (tetraethylmethylenediamine) and
`10% APS (ammonium persulfate) solution were added to the gel
`solution at a final concentration of approximately 0.03%. The
`solution was immediately vacuum siphoned into the capillaries
`and then allowed to polymerize overnight in a cold room. Prior
`to use, both ends of the column were trimmed by about 1 cm and
`
`Image obtained by scanning a four-capillary array. Fluor-
`Figure 3.
`escent primer-labeled M13mp18 DNA “G" fragments were
`loaded In
`100-jzm-i.d. capillaries and then electrophoresed at 240 V/cm (9%
`T, 0% C, 90 mM Trls-borate). The capillary length from the inlet to
`the scan window was 24 cm and the total length was 38 cm. Elec-
`trokinetic sample Injection was performed for 10 s at 9 kV. Each pixel
`represents a 10 pm X 1 s portion of spatial and temporal information.
`then pre-electrophoresed for 30-60 min at 7 kV. The 9% T, 0%
`C gels were sufficiently stable that at least four consecutive DNA
`sequencing separations could be performed.
`Capillary Array Assembly. The capillary array was sand-
`wiched in a capillary holder that is mounted on the translation
`stage. The capillary holder shown in Figure 2 served the dual
`purpose of (1) uniformly constraining each capillary in the array
`to an identical height above the top of the translation stage and
`(2) exposing a small window through which the confocal zone
`probed the capillary interior. Constraining the capillaries to the
`same plane was critical for achieving uniform detection sensitivity
`from each capillary.
`Generation of DNA Sample. Chain-terminated M13mpl8
`DNA fragments were generated using a Sequenase 2.0 sequencing
`kit (United States Biochemical Corp., Cleveland, OH) and a
`fluorescein-tagged primer (FAM, Applied Biosystems, Foster City,
`CA). The detailed procedure has been published elsewhere.26
`Briefly, about 1 pmol of the primer and single-stranded M13mpl8
`DNA were heated to 65 ®C for 3 min and then allowed to cool
`the sequencing extension
`(annealing reaction). Meanwhile,
`mixture was added into a centrifuge tube followed by addition
`of the dideoxy-termination mixture. When the temperature of
`the annealing reaction mixture dropped below 30 °C, a combi-
`
`Agilent Exhibit 1266
`Page 3 of 6
`
`
`
`970 • ANALYTICAL CHEMISTRY, VOL. 64, NO. 8, APRIL 15, 1992
`
`73
`
`1 21
`
`189
`
`269/270
`
`31 4/31 5
`
`473
`
`B
`
`D
`
`5000
`Figure 4. Electropherograms of DNA separation on the four-capillary array. The data in Figure 3 were reduced to a one-dimensional line plot
`by averaging the pixels across the width of each capillary. The base sequence can be read with high signal-to-noise out to greater than 500
`bases beyond the primer. The most intense peaks plateau at the same value because of electronic saturation of the amplifier.
`
`8
`
`si
`
`Figure 5. Expanded view of the Indicated regions from the four-capillary array electropherograms In Figure 4.
`nation of the labeling mixture and diluted enzyme (Sequenase
`other 5 min at 37 °C.
`Instead of adding stop solution, ethanol
`2.0) were added, and the mixture was incubated for 5 min at room
`precipitation was immediately used to terminate the reaction and
`temperature. This mixture was
`the DNA sequencing sample. The high concentration of
`then transferred to the tube
`recover
`having the termination mixture and allowed to incubate for an-
`conductive ions present in the DNA sequencing sample after the
`
`Agilent Exhibit 1266
`Page 4 of 6
`
`
`
`termination step would reduce the amount of DNA that can be
`loaded into each capillary by electrokinetic injection. To coun-
`teract this effect, ethanol precipitation was performed on all DNA
`of 80% (v/v) formamide
`samples followed by resuspension in 6
`to give a concentration about 10-fold higher than that used in
`slab gels. The sample was heated at 90 °C for 3 min to ensure
`denaturation and then placed on ice until sample injection.
`Sample Introduction.
`The DNA sequencing sample was
`placed in a 500-centrifuge tube for electrokinetic injection.
`The same electric field strength (240 V/cm) used during separation
`was also applied during sample injection. The typical injection
`time was 10 s. After injection, the inlets of the capillaries were
`removed from the centrifuge tube and placed into a reservoir filled
`with fresh buffer.
`RESULTS AND DISCUSSION
`Figure 3 presents an image obtained from on-line confocal
`scanning of a four-capillary array during electrophoresis of
`a mixture of DNA sequencing fragments. The horizontal
`direction is the physical dimension representing the geometric
`arrangement of the capillary array while the vertical direction
`is temporal representing the passage of fluorescent DNA
`fragments through the detection window. For lane-to-lane
`comparison, identical samples of “G" base DNA fragments
`were simultaneously electrokinetically injected into each ca-
`pillary. The overall elapsed data acquisition time is ~80 min
`after passage of the primer. An expanded region of the image
`is presented in Figure 3. The bands in all four lanes are well
`resolved, and the resolution extends throughout the se-
`quencing run with sufficient signal-to-noise to detect bands
`than 500 bases beyond the primer.
`more
`Figures 4 and 5 present line plots of the DNA signal inte-
`the width of each capillary. A signal-to-noise
`grated across
`ratio of approximately 20 is observed out to base 385 (~65
`min) and bands are detected out to base 500 with the present
`experimental conditions. The number of theoretical plates
`is >1.9 X 106 (at base 385) over
`a 24-cm effective column
`length.
`A comparison was made between the signal-to-noise ratio
`obtained in the scanning mode and the case where the system
`is focused in the center of a single stationary capillary. The
`latter approach is analogous to traditional on-column detection
`from a single capillary. The sensitivity limits extrapolated
`for the scanning mode were found to be ~2 X 1CT12 M (S/N
`= 3) by flowing 1 X 10'11 M fluorescein through an open
`capillary. The sensitivity limits for the stationary mode were
`found to be ~1 X 10'12 M indicating that scanning does not
`seriously degrade the signal-to-noise ratio. These detection
`limits are at least as good as those reported from single cap-
`illaries using the conventional 90° detection geometry.10 The
`background from the gel-filled capillaries was ~2.6 times (n
`= 4) higher than that from a capillary filled with just TBE
`buffer. Thus, the presence of the gel increased the background
`noise by a factor of ~ 1.6.
`This work indicates that the overall throughput perform-
`ance of CAE can be very high. Figures 3-5 show that satis-
`factory sequencing information is obtained out to 500 bases
`for each of four capillaries in less than 2 h. An important issue
`is determining how feasible it is to increase the size of our
`capillary arrays. Figure 6 presents the results of a DNA
`sequencing fragment separation performed using an array of
`24 capillaries. The resolution, signal-to-noise, and background
`are uniformly good for all the capillaries in the array, dem-
`onstrating that extrapolation to larger numbers of capillaries
`is feasible. The limiting throughput of CAE using our system
`depends upon the total number of capillaries, N, that can be
`scanned. The equation, N = vT/2D, defines how N depends
`on the scan speed (a), the scan repetition period (T), and the
`capillary outside diameter (D). For example, we can run 100
`200-/im-o.d. capillaries using a scan rate of 4 cm/s and a l-s
`
`ANALYTICAL CHEMISTRY, VOL. 64, NO. 8, APRIL 15, 1992 • 971
`
`10
`
`15
`
`20
`
`24
`
`26
`
`105
`
`- 248
`
`Image obtained by scanning a 24-capllary array. The DNA
`Figure 6.
`sample consisted of fluorescent primer-labeled M13mp18 T-fragments.
`All other conditions were
`the same as those in Figure 3.
`
`Increasing the array size would require
`scan repetition period.
`(1) an increase in the scan speed, (2) the use of smaller o.d.
`capillaries, and (3) an increase in the scan repetition period
`which would reduce the temporal resolution of the electro-
`phoretic separation. Since reliable scan systems have velocities
`up to 10 cm/s and capillaries with o.d.’s of 150 nm are com-
`mercially available, a limit of approximately 330 capillar-
`ies/array can be projected assuming a l-s scan repetition
`period.
`Finally, it should be noted that there is a difference in the
`migration time of a given DNA band from capillary-to-ca-
`pillary. This may be caused by inhomogeneities of the gel
`matrix or the presence of local nonuniform variations in the
`It has previously been estimated that
`electric field strength.
`there is a 5% variation in migration time between identical
`samples on different gel columns.4 Table I lists the migration
`time for selected DNA fragments from each capillary in the
`four-capillary array, and a similar variation in migration time
`is observed.
`The velocity shift of the DNA bands from capillary-to-ca-
`pillary may preclude sequencing DNA with CAE using a single
`fluorophore and four different capillaries, one for each base.
`For DNA sequencing, the present apparatus must be ex-
`panded to a multicolor detection system to sequence all four
`
`Agilent Exhibit 1266
`Page 5 of 6
`
`
`
`972 • ANALYTICAL CHEMISTRY, VOL. 64, NO. 8, APRIL 15, 1992
`
`Table I. Migration Time of Selected DNA Fragments in
`the Four-Capillary Array
`migration time (s)
`column 2
`column 3
`
`column 4 % CV
`
`base
`number
`
`column 1
`
`87
`144
`185
`232
`285
`335
`385
`
`1308
`1734
`2069
`2594
`3129
`3621
`4168
`
`1286
`1729
`2065
`2454
`2891
`3300
`3679
`
`1283
`1808
`2166
`2448
`2884
`3287
`3658
`
`1337
`1764
`2112
`2583
`3079
`3499
`3902
`
`1.9
`2.2
`2.5
`3.2
`4.2
`4.7
`6.2
`
`bases in a single capillary. Four-color detection schemes have
`been developed for single capillaries8 and for slab gels.19 We
`have recently introduced a multicolor confocal fluorescence
`scanner and used it for DNA detection in slab gels.26 The
`successful application of this multicolor fluorescence scanner
`to the detection of capillary arrays should provide a valuable
`new sequencing technology.
`In summary, we have demonstrated that it is possible to
`perform high-sensitivity fluorescence detection of capillary
`arrays by using a confocal fluorescence scanner. The most
`important advantage of this approach is that multiple samples
`can be easily loaded and rapidly separated in parallel followed
`by high-sensitivity detection. The use of capillary arrays
`potentially resolves the throughput problems that limit the
`utility of CE in, for example, DNA sequencing.29 In addition,
`CAE provides an opportunity for the large-scale optimization
`of analytical separations. Commercially made capillary arrays
`could be constructed for large-scale parallel sample intro-
`duction. CAE should be a valuable new technique for rapid,
`parallel separation and analysis.
`ACKNOWLEDGMENT
`We thank Alexander Glazer
`for valuable discussions
`throughout the course of this work and Jiun-Wei Chen for
`helpful suggestions on the preparation of non-cross-linked
`poly(acrylamide) gel columns and DNA sequencing samples.
`This research was supported by the Director, Office of Energy
`Research, Office of Health and Environmental Research of
`the U.S. Department of Energy, under Contract DE-FG-
`91ER61125. X.H. was supported as a DOE Human Genome
`Distinguished Postdoctoral Fellow during the course of this
`research.
`
`REFERENCES
`(1) Jorgenson, J. W.; Lukacs, K. D. Science 1983, 222, 266-272.
`(2) Gordon, M. J.; Huang, X.; Pentoney, S. L., Jr.; Zare, R. N. Science
`1988, 242, 224-228.
`(3) Ewing, A. G.; Wallingford, R. A.; Oteflrowicz, T. M. Anal. Chem. 1989,
`61, 292A-303A.
`(4) Karger, B. L.; Cohen, A. S.; Guttman, A. J. Chromatogr. 1989, 492,
`585-614.
`(5) Kuhr, W. G. Anal. Chem. 1990, 62, 403R-414R.
`(6) Cohen, A. S.; Najarian, D. R.; Paulus, A.; Guttman, A.; Smith, J. A.;
`Karger, B. L. Proc. Natl. Acad. Scl. U.S.A. 1988, 85, 9660-9663.
`(7) Heiger, D. N.; Cohen, A. S.; Karger, B. L. J. Chromatogr. 1990, 516,
`33-48.
`(8) Luckey, J. A.; Drossman, H.; Kostichka, A. J.; Mead, D. A.; D'Cunha,
`J.; Norris, T. B.; Smith, L. M. Nucleic Acids Res. 1990,
`18,
`4417-4421.
`(9) Swerdlow, H.; Wu, S.; Harke, H.; Dovlchi, N. J. J. Chromatogr. 1990,
`516, 61-67. Swerdlow, H.; Zhang, J. Z.; Chen, D. Y.; Harke, H. R.;
`Grey, R.; Wu, S.; Dovlchi, N. J. Anal. Chem. 1991, 63, 2835-2841.
`(10) Swerdlow, H.; Gesteland, R. Nucleic Adds Res. 1990,
`18,
`1415-1419.
`(11) Drossman, H.; Luckey, J. A.; Kostichka, A. J.; D'Cunha, J.; Smith, L.
`M. Anal. Chem. 1990, 62, 900-903.
`(12) Compton, S. W.; Brownlee, R. G. BloTechnlques 1988, 6, 432-439.
`(13) Cohen, A. S.; Najarian, D.; Smith, J. A.; Karger, B. L. J. Chromatogr.
`1988, 458, 323-333.
`(14) Cheng, Y.-F.; Dovlchi. N. J. Science 1988, 242, 562-564.
`(15) Hjerten, S.; Elenbring, K.; Kilar, F.; Liao, J.-L.; Chen, A. J. C.; Slebert,
`C. J.; Zhu, M.-O. J. Chromatogr. 1987, 403, 47-61.
`(16) Gassmann, E.; Kuo, J. E.; Zare, R. N. Science 1985, 230, 813-814.
`(17) Ansorge, W.; Sproat, B.; Stegemann, J.; Schwager, C.; Zenke, M. Nu-
`cleic Adds Res. 1987, 15, 4593-4602.
`(18) Brumbaugh, J. A.; Middendorf, L. R.; Grone, D. L.; Ruth, J. L. Proc.
`Natl. Acad. Scl. U.S.A. 1988, 85, 5610-5614.
`(19) Smith, L. M.; Sanders, J. Z.; Kaiser, R. J.; Hughes, P.; Dodd, C.; Con-
`nell, C. R.; Heiner, C.; Kent, S. B. H.; Hood, L. E. Nature 1986, 321,
`674-679.
`(20) Zagursky, R. J.; McCormick, R. M. BloTechnlques 1990, 9, 74-79.
`(21) Prober, J. M.; Trainor, G. L.; Dam, R. J.; Hobbs, F. W.; Robertson, C.
`W.; Zagursky, R. J.; Cocuzza, A. J.; Jensen, M. A.; Baumeister, K.
`Science 1987, 238, 336-341.
`(22) Glazer, A. N.; Peck, K.; Mathles, R. A. Proc. Natl. Acad. Scl. U.S.A.
`1990, 87, 3851-3855.
`(23) Mathles, R. A.; Peck, K.; Stryer, L. In Bioimaging and Two-Dimension-
`al Spectroscopy, Smith, L. C„ Ed.; SPIE-The International Society for
`Optical Engineering; Bellingham, WA, 1990; pp 52-59.
`(24) Rye, H. S.; Quesada, M. A.; Peck, K.; Mathles, R. A.; Glazer, A. N.
`Nucleic Adds Res. 1991, 19, 327-333.
`(25) Quesada, M. A.; Rye, H. S.; Gingrich, J. C.; Glazer, A. N.; Mathles, R.
`A. BloTechnlques 1991, 10, 616-625.
`(26) Rye, H. S.; Yue, S.; Quesada, M. A.; Haugland, R. P.; Mathles, R. A.;
`Glazer, A. N. In Methods In Enzymoiogy, Recombinant DNA. Part H\
`Wu, R. Ed.; in press.
`(27) Hernandez, L.; Marquina, R.; Escalona, J.; Guzman, N. J. Chromatogr.
`1990, 502, 247-255.
`(28) Hernandez, L.; Escalona, J.; Joshi, N.; Guzman, N. A. J. Chromatogr.
`1991, 559, 183-196.
`(29) Smith, L. M. Nature 1991, 349, 812-813.
`Received for review November 8,1991. Accepted January
`21, 1992.
`
`Agilent Exhibit 1266
`Page 6 of 6
`
`
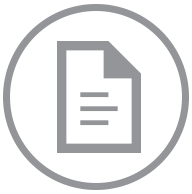
Accessing this document will incur an additional charge of $.
After purchase, you can access this document again without charge.
Accept $ ChargeStill Working On It
This document is taking longer than usual to download. This can happen if we need to contact the court directly to obtain the document and their servers are running slowly.
Give it another minute or two to complete, and then try the refresh button.
A few More Minutes ... Still Working
It can take up to 5 minutes for us to download a document if the court servers are running slowly.
Thank you for your continued patience.
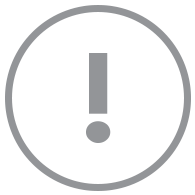
This document could not be displayed.
We could not find this document within its docket. Please go back to the docket page and check the link. If that does not work, go back to the docket and refresh it to pull the newest information.
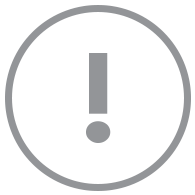
Your account does not support viewing this document.
You need a Paid Account to view this document. Click here to change your account type.
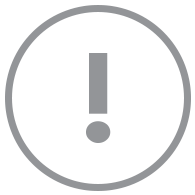
Your account does not support viewing this document.
Set your membership
status to view this document.
With a Docket Alarm membership, you'll
get a whole lot more, including:
- Up-to-date information for this case.
- Email alerts whenever there is an update.
- Full text search for other cases.
- Get email alerts whenever a new case matches your search.
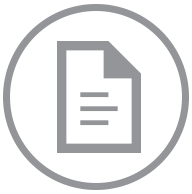
One Moment Please
The filing “” is large (MB) and is being downloaded.
Please refresh this page in a few minutes to see if the filing has been downloaded. The filing will also be emailed to you when the download completes.
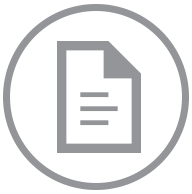
Your document is on its way!
If you do not receive the document in five minutes, contact support at support@docketalarm.com.
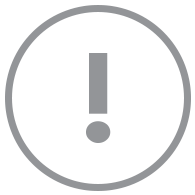
Sealed Document
We are unable to display this document, it may be under a court ordered seal.
If you have proper credentials to access the file, you may proceed directly to the court's system using your government issued username and password.
Access Government Site